Light Absorption and Scattering
When sunlight enters water, the blue and violet wavelengths, which are shorter and scatter more efficiently, penetrate deeper. In contrast, longer wavelengths, such as red, orange, and yellow, are absorbed more quickly. At the molecular level, water absorbs these longer wavelengths first, leaving blue and violet to be scattered and reflected. This scattering disperses the shorter wavelengths more effectively, creating the deep blue appearance.
The physical size of water molecules plays a crucial role here. These molecules are small enough to scatter short wavelength light more than they scatter longer wavelength light, similar to how the gases in our atmosphere scatter blue light, making the sky appear blue.
As light penetrates further, the absorption process continues. Red and orange light vanish within a few meters. Yellow and green follow shortly after, leaving predominantly blue and violet light. This results in the characteristic blue hue we associate with ocean waters.
Not all bodies of water appear the same shade of blue. Shallow waters often look turquoise due to the light reflecting off sandy ocean floors. In contrast, deeper waters absorb more light, turning darker shades of blue. Similarly, sediments or particles in the water can alter the perceived color. For instance, after a storm or in a muddy river, you might notice a brownish tint, caused by clouds of particles scattering light differently.
Phytoplankton, tiny plant-like organisms, also have a significant impact. These organisms contain chlorophyll, which absorbs red and blue light but reflects green light. In areas with abundant phytoplankton, the water can appear greenish due to this reflected light.
This selective absorption and scattering process isn't confined to the surface. As you go deeper, the principles remain the same but become more pronounced. With each descending meter, more of the light spectrum gets absorbed, and the water appears progressively darker. By the time you reach depths where no sunlight can penetrate, the environment plunges into darkness. The only sources of light are bioluminescent organisms that produce their own light through chemical reactions.
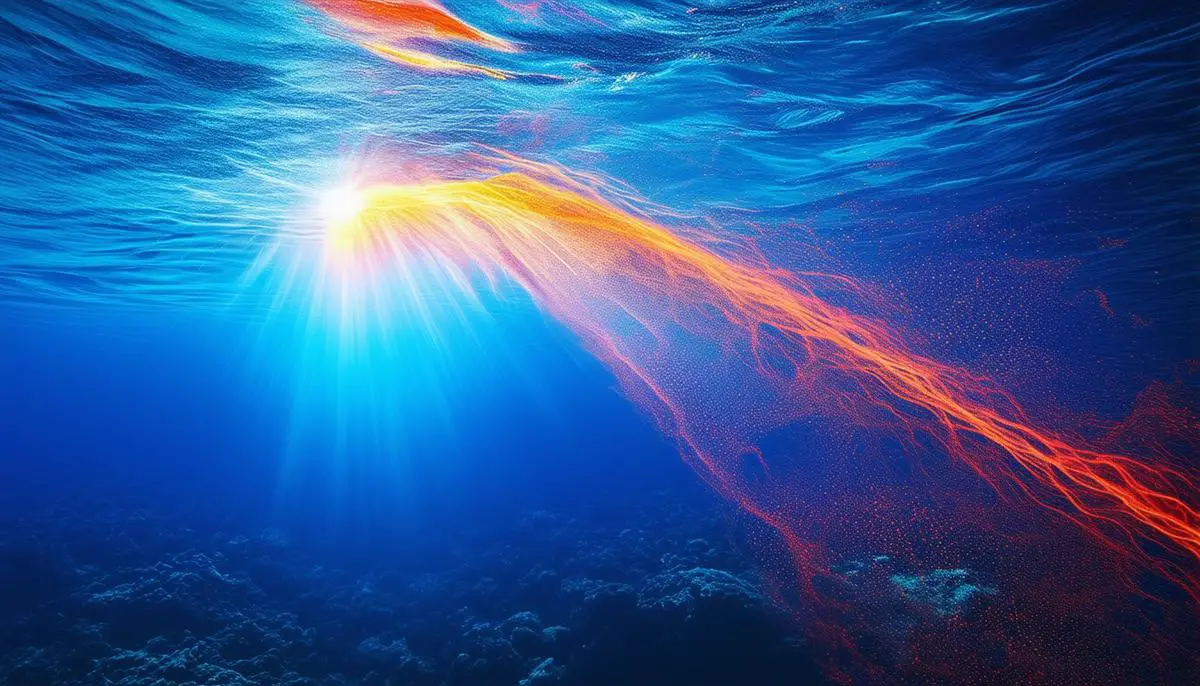
Rayleigh Scattering
Rayleigh Scattering is a fundamental concept that explains much about the color of our natural world, from the blue sky to the blue hue of the oceans. Named after Lord Rayleigh, the scientist who first described it in the 19th century, Rayleigh scattering occurs when light waves interact with particles that are much smaller than the wavelength of the light itself. This scattering is far more effective at shorter wavelengths — the blue and violet ends of the spectrum.
When sunlight, which is composed of multiple colors, enters the Earth's atmosphere, it encounters numerous small particles, including nitrogen and oxygen molecules. Shorter wavelengths, such as blue and violet light, are scattered in all directions by these particles more efficiently than longer wavelengths like red and yellow. Despite violet light being scattered even more than blue, our eyes are less sensitive to violet and more to blue, thus the sky primarily appears blue to us.
Interestingly, this same principle extends underwater, where similar scattering mechanisms operate. In the aquatic environment, the molecules of water are the primary scatterers. When sunlight penetrates the surface of the ocean, it again encounters water molecules that preferentially scatter shorter wavelengths. This scattering process ensures that blue light, due to its shorter wavelength, is dispersed throughout the water more effectively than longer wavelengths.
The efficiency of Rayleigh scattering increases inversely with the fourth power of the wavelength of light. In simpler terms, shorter wavelengths are scattered approximately 1/λ4 times more than longer wavelengths. As a result, in a medium like water, where scattering isn't as strong as in the air, the relative proportion of scattered blue light increases compared to longer wavelengths, enriching the blue appearance we observe.
This scattering becomes especially noticeable in clearer, purer water, where there are fewer particles and impurities to reflect other wavelengths of light. In these conditions, the ocean reflects and scatters predominantly blue light, creating the vast blue vistas we're familiar with. The sensitivity of our eyes to blue light compounds this effect, making the blue scattering even more apparent.
On a molecular level, water's unique properties further impact the behavior of light. The small size of water molecules means they are excellent at scattering shorter wavelengths of light. As you travel deeper into the ocean, the blue light that is scattered becomes one of the few colors that remains, because it is scattered repeatedly within the water rather than being absorbed. This scattering maintains the blue hue at various depths, whereas longer wavelengths like red and green are absorbed quickly and do not penetrate as deeply.
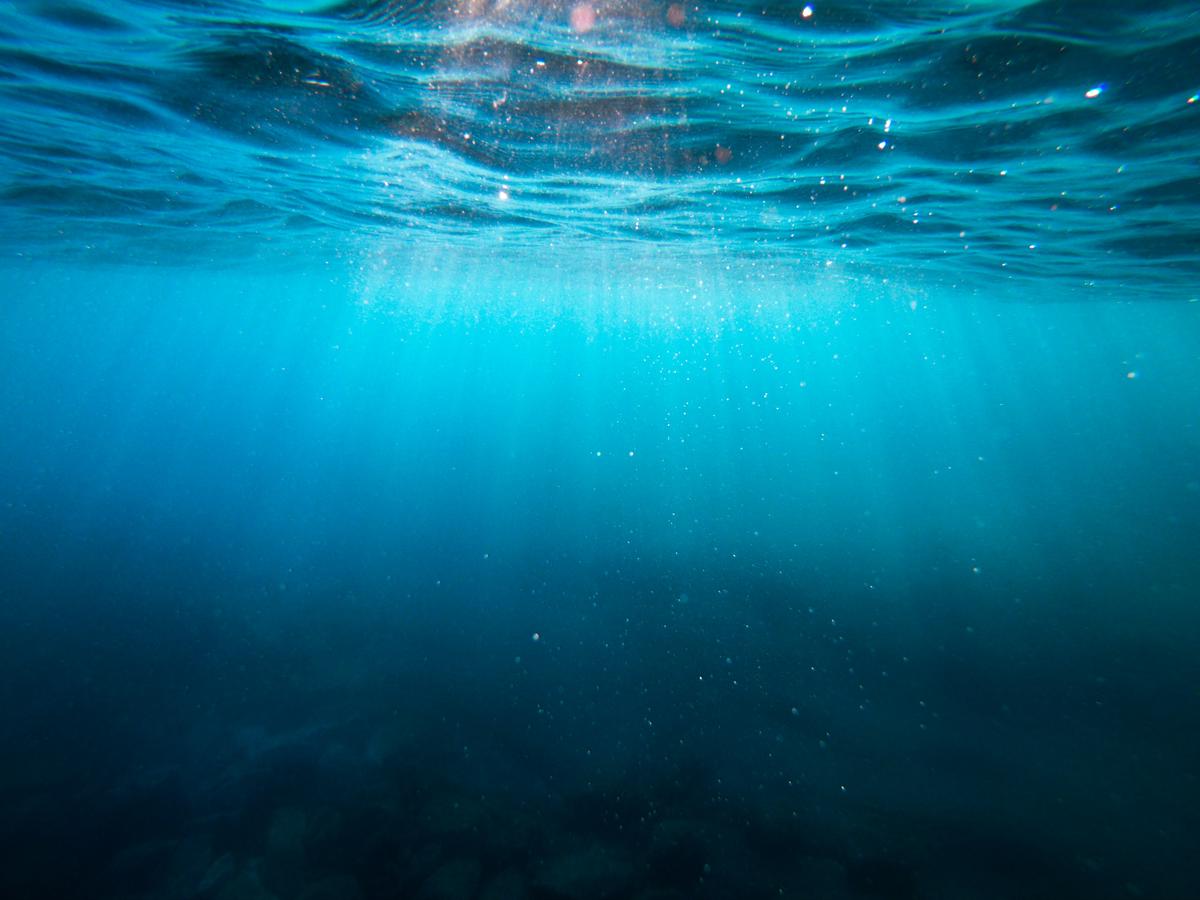
Photo by cristianpalmer on Unsplash
Variations in Ocean Color
The ocean's color is far from uniform, and its shades can range from deep navy to vibrant turquoise, and even green or brown. This variability in ocean color is primarily influenced by water depth, the presence of various particles or organisms like phytoplankton, and geographical or environmental factors.
One of the most striking variations is observed in shallow coastal waters, which often exhibit a lighter, more turquoise color. This is due to the fact that in these regions, sunlight can penetrate to the ocean floor, which then reflects light back upwards. The sand and other materials at the bottom of shallow waters contribute to this reflection, enhancing the turquoise hue.
In contrast, deeper ocean waters absorb more light. As sunlight penetrates these depths, the longer wavelengths of light, like red, orange, and yellow, are absorbed relatively quickly, leaving predominantly the shorter blue wavelengths. This explains why deep ocean waters appear to be a darker blue or even navy. The further the depth, the more pronounced this effect, as almost all light is absorbed and only minimal blue light remains.
The presence of particles and organisms in the water also significantly influences its color:
- Phytoplankton contain chlorophyll and other pigments that absorb certain wavelengths of light and reflect others. Chlorophyll absorbs red and blue wavelengths efficiently but reflects green light, giving regions rich in phytoplankton a distinctive greenish tint. This is often why coastal waters, where nutrients are plentiful, can appear green.
- Sediments from rivers or stirred-up ocean floors during storms scatter light differently. When water contains significant amounts of these particles, the color can shift towards brown or murky hues. For example, runoff from rivers may carry sediments that cloud the water, scattering more light and giving it a brownish appearance. This effect is often pronounced after heavy rains or in areas with strong currents that stir up the ocean floor.
Geographical and environmental factors further complicate the color palette of the ocean. Coral reefs and underwater vegetation can also play roles in the reflected colors. Areas with abundant coral reefs often reflect a variety of colors, including greens and blues, increasing the visual intricacy of these waters.
Environmental conditions, such as algal blooms, can cause drastic changes in water color. These blooms, a rapid increase in the population of algae, often make the water appear green, red, or even brown due to the high concentration of pigments produced by the algae. This affects the ecosystem and is also a visible marker of changes in nutrient levels and water quality.
In tropical and subtropical regions, the vibrant blue and turquoise waters are often due to the low content of organic matter and minimal suspended particles, allowing for clearer water that reflects and scatters light in ways that highlight the blue spectrum. Conversely, in polar regions, the water may appear darker due to the dissolved organic matter and less intense sunlight infiltration.
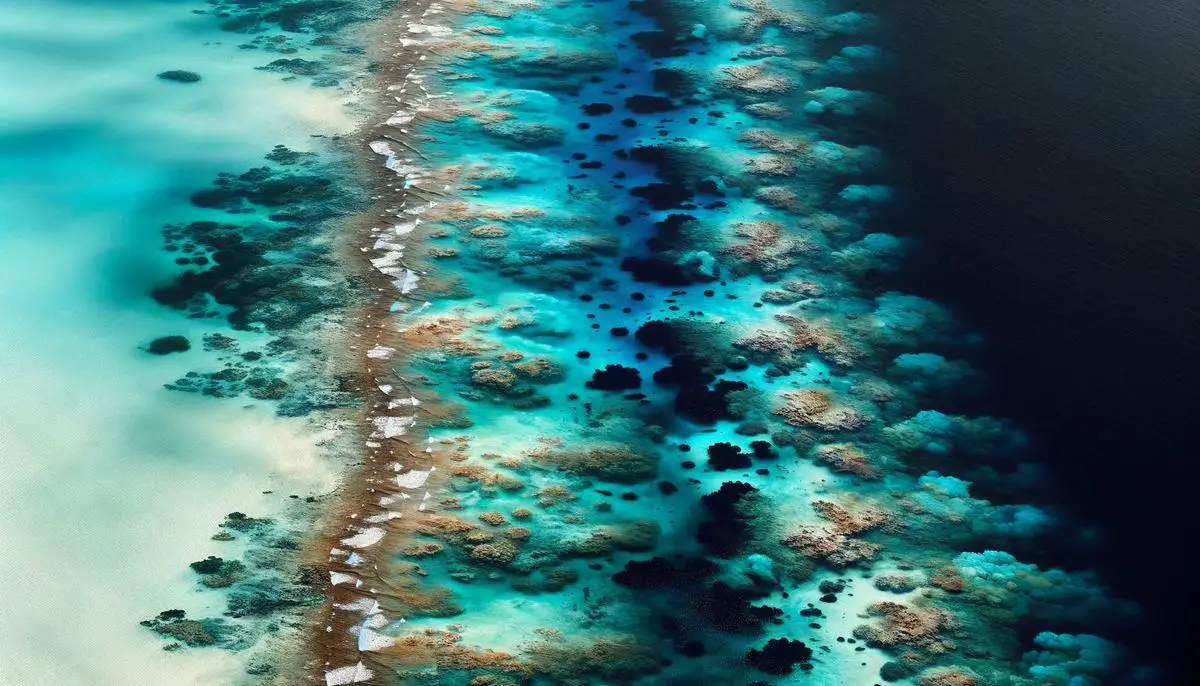
Scientific Studies and Environmental Implications
Scientists use the color of the ocean as a vital indicator to monitor phytoplankton populations and assess the overall health of marine ecosystems. These tiny plant-like organisms play a crucial role in oceanic and global ecological health.1 By analyzing the subtle variations in ocean color from satellite imagery and direct water sampling, researchers can gain insights into the density and distribution of these microscopic life forms.
Phytoplankton, the primary producers in the ocean, operate much like terrestrial plants, using photosynthesis to convert sunlight and carbon dioxide into energy. This process supports marine food webs and significantly influences global carbon cycles. As phytoplankton photosynthesize, they absorb carbon dioxide from the atmosphere, helping to mitigate the effects of climate change.2 This carbon is then transferred through the food web or sinks to the ocean floor, sequestering it for long periods.
The presence and concentration of phytoplankton alter the water's color due to the chlorophyll and other pigments they contain. By reflecting green light and absorbing other wavelengths, particularly reds and blues, phytoplankton-rich regions exhibit distinct color signatures detectable from space. Satellites equipped with spectroradiometers measure the ocean's color across different wavelengths, providing data that allows scientists to estimate phytoplankton concentrations and their chlorophyll content.
This remote-sensing technology is invaluable. It permits continuous, global-scale monitoring of the oceans, revealing seasonal and interannual variations in phytoplankton populations. For instance, by tracking chlorophyll-a concentrations, scientists can identify and predict algal blooms, which are critical for managing fisheries, protecting coastal waters, and ensuring the safety of consuming seawater-derived food products.3 These blooms, often visible as green or even reddish patches in the ocean, can indicate nutrient overloads and potential toxin production, affecting marine life and human health.
In addition to detecting phytoplankton abundance, studying ocean color assists in assessing the broader health of marine ecosystems. Healthy phytoplankton populations suggest robust primary production, supporting higher trophic levels including fish, marine mammals, and seabirds. Conversely, declining phytoplankton can signal problems such as nutrient depletion or increased water temperatures, which can disrupt marine food webs and biodiversity.
Environmental and climatic factors heavily influence phytoplankton dynamics. Ocean color studies help scientists understand how climate change affects these dynamics. Rising sea temperatures, ocean acidification, and changes in nutrient availability caused by shifting ocean currents and melting polar ice all impact phytoplankton growth rates and distribution.4 For example, warmer temperatures can lead to stratification in the ocean, reducing nutrient mixing from the deeper waters to the surface and potentially lowering phytoplankton productivity.
Moreover, phytoplankton's role in carbon sequestration underscores their importance in the fight against global climate change. As phytoplankton absorb carbon dioxide for photosynthesis, they play a part in reducing greenhouse gases in the atmosphere. When phytoplankton die, they sink, carrying the carbon they have absorbed to the ocean floor. This process, known as the biological carbon pump, is a critical component in regulating the Earth's carbon balance and storing carbon away from the atmosphere for centuries.
Understanding the health of marine ecosystems through ocean color helps gauge marine biodiversity and enhances our predictive capabilities regarding global ecological balance. It facilitates more informed environmental policies and conservation strategies to combat climate change and protect marine resources.
By continuing to refine our ability to analyze ocean color, scientists can better track changes in phytoplankton populations, investigate the health of our oceans, and develop strategies to maintain the delicate balance of our planet's ecosystems. This line of research underscores the interconnectedness of terrestrial and marine environments and how pivotal maintaining marine health is to the stability of Earth's overall climate system.
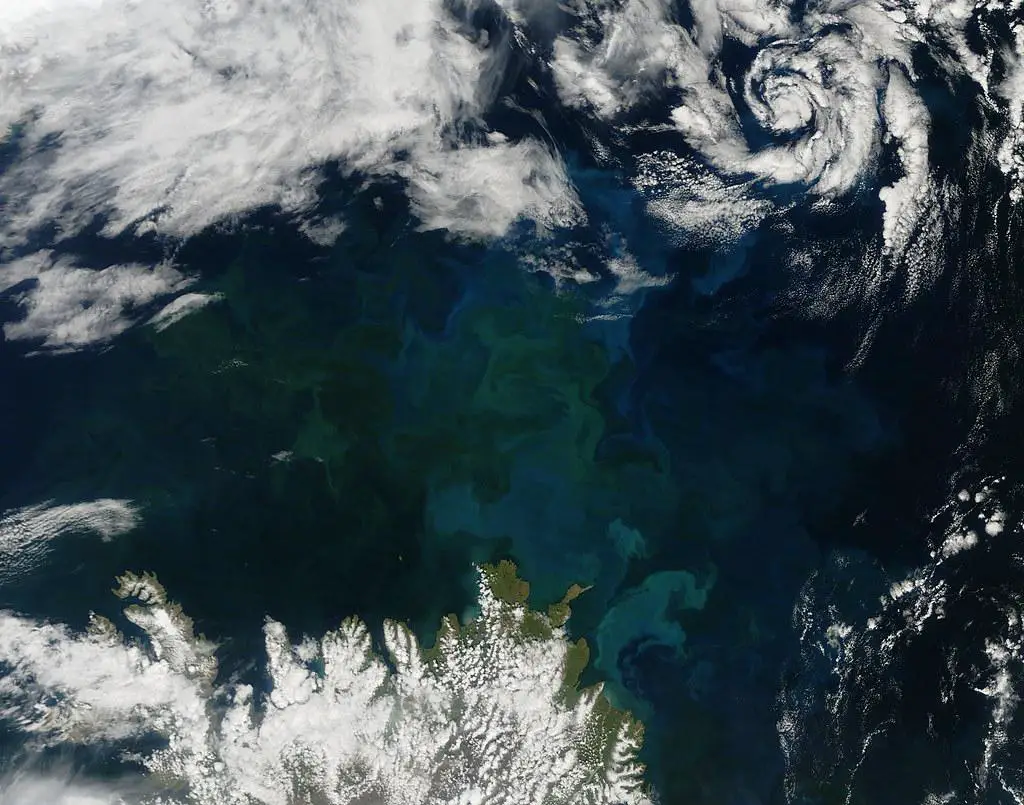
- Field CB, Behrenfeld MJ, Randerson JT, Falkowski P. Primary production of the biosphere: integrating terrestrial and oceanic components. Science. 1998;281(5374):237-240.
- Falkowski PG, Barber RT, Smetacek V. Biogeochemical controls and feedbacks on ocean primary production. Science. 1998;281(5374):200-206.
- McClain CR. A decade of satellite ocean color observations. Annual Review of Marine Science. 2009;1:19-42.
- Sarmiento JL, Slater R, Barber R, et al. Response of ocean ecosystems to climate warming. Global Biogeochemical Cycles. 2004;18(3).