Heliosphere and Heliopause
The Sun's influence extends far beyond the orbits of the planets, creating a magnetic bubble known as the heliosphere. This massive region surrounds our solar system, sculpted and molded by the solar wind – a continuous flow of charged particles emanating from the Sun. The heliosphere acts as a protective shield against the harsh environment of interstellar space, with its boundary marked by the heliopause.
The heliopause is where the solar wind slows to a halt, meeting the interstellar medium. Here, interactions between the solar magnetic field and the external cosmic forces play out. Contrary to a clear demarcation, this region is thick and complex, more like a twilight zone. Voyager 1's journey through the heliopause was marked by sudden and significant changes in particle densities and plasma waves. It crossed this boundary on August 25, 2012, at a distance of about 121 astronomical units (AU) from the Sun.1
Voyager 1's instruments detected distinct characteristics marking its foray into interstellar space. Surprisingly, magnetic field readings showed little change in direction. Researchers didn't observe the anticipated shifts, suggesting that the connection between the Sun's magnetic field and interstellar fields might be more complicated than previously thought. Intriguingly, galactic cosmic rays penetrated through, revealing a porous heliopause, contradicting earlier expectations.
Currently sailing through interstellar space, Voyager 1 provides first-hand glimpses of this uncharted territory. It confirms that the interstellar medium is influenced by solar activities, even against the backdrop of an otherwise distant cosmos. Continuous updates from Voyager 1 showcase a dynamic interplay of forces far beyond our habitual cosmic neighborhood.
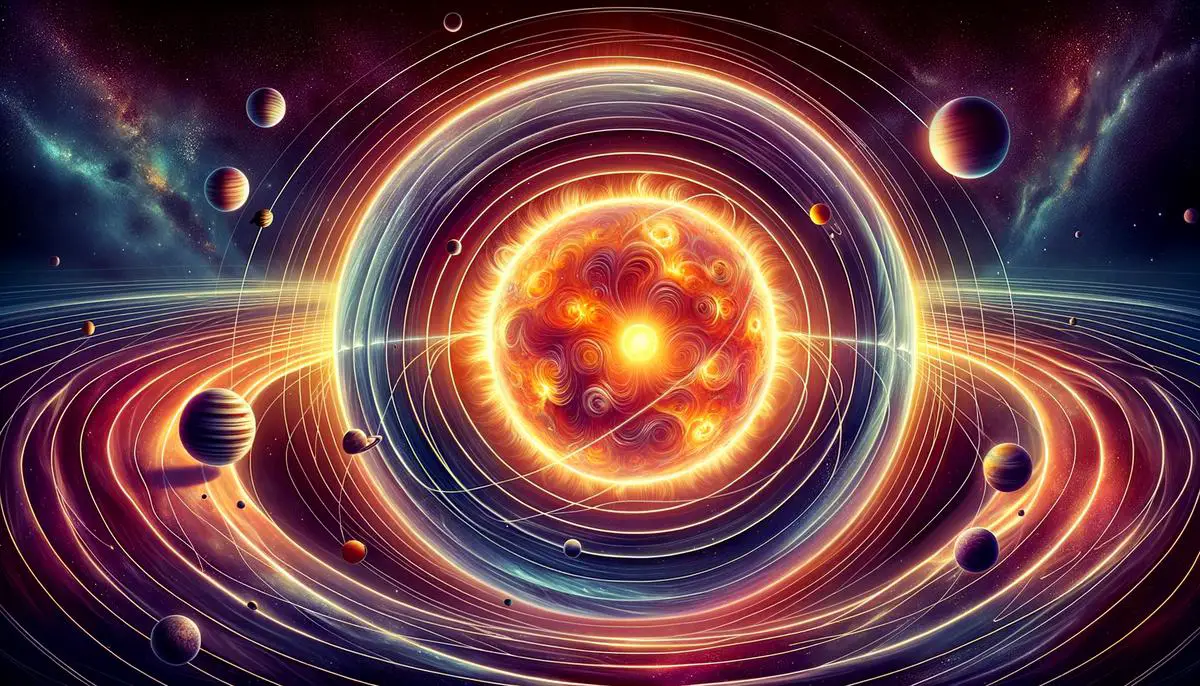
Voyager 1's Journey So Far
Launched on September 5, 1977, Voyager 1 embarked on its epic journey from Cape Canaveral, Florida. As part of NASA's ambitious Voyager program, it aimed to study the outer planets—Jupiter and Saturn—and their moons. Its twin, Voyager 2, launched just 16 days earlier, but Voyager 1 would ultimately accelerate ahead, making more rapid progress along its path.
Voyager 1's notable encounters began with Jupiter in March 1979. The probe provided humanity with the first close-up images of the gas giant and its moons, revealing fascinating details about their geology and atmospheres. Among its numerous discoveries, one of the most surprising was the volcanic activity on Io, one of Jupiter's moons. The data sent back from Voyager 1 confirmed active volcanoes, making Io the most volcanically active body in the solar system.2 These insights dramatically broadened our understanding of planetary science.
Continuing its journey, Voyager 1 reached Saturn in November 1980. The spacecraft offered unprecedented insights into the ringed planet, including:
- Identifying complex structures within its rings
- Gaining information about Titan, Saturn's largest moon, and its thick, nitrogen-rich atmosphere
- Sparking curiosity about the possibility of liquid bodies on Titan's surface
Following its Titan flyby, a crucial maneuver occurred: Voyager 1 used a gravity assist from Saturn to alter its trajectory, sending it on a path out of the ecliptic plane and to the outermost reaches of our solar system. This changed Voyager 1's mission focus from planetary encounters to pioneering the study of the solar system's boundary and beyond.
As Voyager 1 continued to drift away from the Sun, it approached a region where solar influence waned, and the vastness of interstellar space began. This boundary was the heliopause, a term that would grow in significance thanks to Voyager's discoveries. By late 2012, Voyager 1 recorded a dramatic increase in plasma density, indicating that it had left the heliosphere and entered interstellar space. This historic crossing made Voyager 1 the first human-made object to traverse into the domain between stars.
Since then, Voyager 1 has been sending back invaluable data about the interstellar medium. The spacecraft continues to surprise scientists with findings about the magnetic field direction and particle densities just beyond the heliopause.
Presently, Voyager 1 is over 14 billion miles from Earth. It continues its journey at over 38,000 miles per hour, relentlessly advancing to the Oort Cloud. Despite the immense distance, the spacecraft remains a silent ambassador, conducting unprecedented research in a realm where no other probe has ventured.
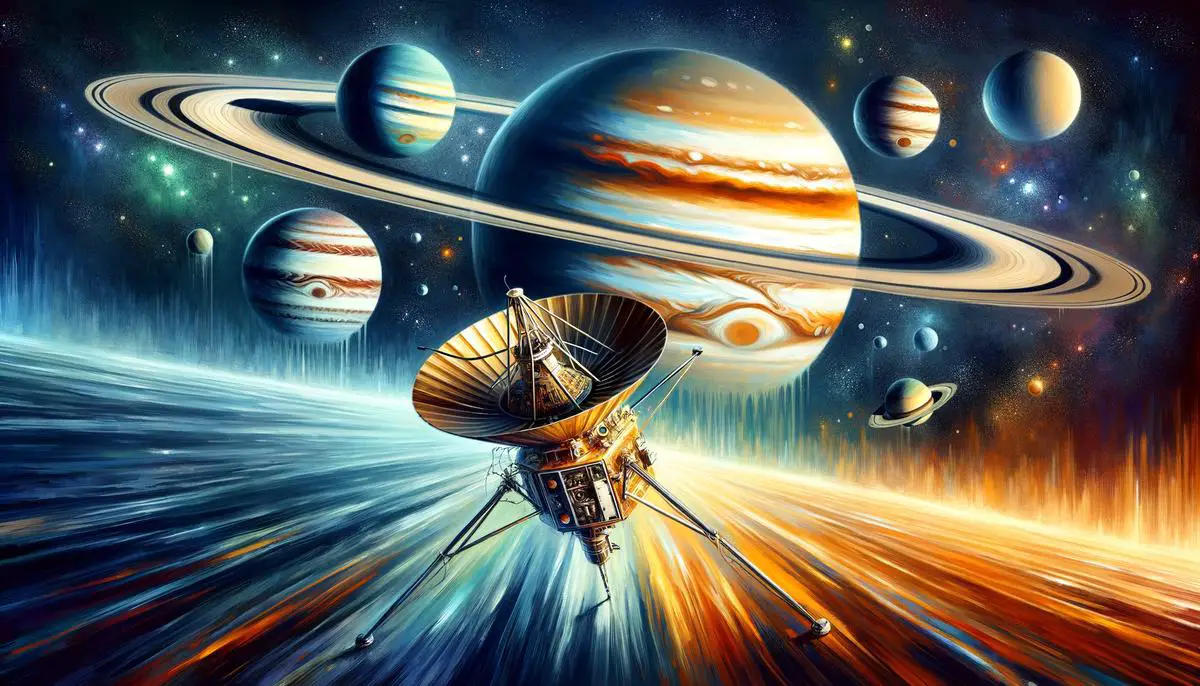
Interstellar Medium and Its Characteristics
As Voyager 1 journeys deeper into the interstellar medium, it unveils an environment drastically different from the heliosphere. The interstellar medium consists of sparse particles, predominantly hydrogen and helium gases, and cosmic rays, interspersed with dust. This environment, while incredibly thin compared to the relative density of Earth's vicinity, is teeming with mysteries and unexpected phenomena.
One of the primary indicators that Voyager 1 had entered interstellar space was the observed change in particle density. As it crossed the heliopause, the spacecraft detected a sudden increase in plasma density, a stark contrast to the lower density within the heliosphere. Researchers noted that the density of electrons — a crucial component of plasma — was about 40 times greater in the interstellar medium than within the solar bubble, marking a remarkable transition.3
Equally intriguing were the findings regarding the magnetic fields. Prior expectations suggested a noticeable shift in the direction of the magnetic field once Voyager 1 passed the heliopause. Surprisingly, data showed that the magnetic field outside the heliosphere aligned closely with that inside, challenging preconceived models. This anomaly suggests that the interface between the solar magnetic field and interstellar fields is far more intricate, potentially involving magnetic reconnection or other poorly understood mechanics that blend the solar and galactic influences.
Beyond these foundational observations, Voyager 1's instruments have detected variations in cosmic rays and space weather. The heliosphere's boundaries appeared somewhat porous; Voyager recorded an influx of galactic cosmic rays and traces of solar particles leaking through. This permeability indicates a dynamic interaction zone rather than a rigid barrier, reshaping our paradigm of how solar and interstellar environments interact.
The influence of solar activity extends surprisingly far into interstellar space. Coronal mass ejections (CMEs) and other solar events propagate through the heliopause, altering the interstellar medium's particle density and magnetic field structure even billions of miles from the Sun. These interactions imply an unseen tethering of our solar system's phenomena to the distant regions of the cosmos, illustrating an enduring connection amid apparent detachment.
Voyager 1's journey through this area continues to yield significant revelations. It discovered that galactic cosmic rays exhibit directional preferences tied to the solar magnetic field, opposing the expectation of an isotropic distribution. This directional behavior correlates with solar cycles and disturbances, further exemplifying the interconnectedness of cosmic scales.
Such findings elevate our understanding of the interstellar medium as a vibrant arena influenced by both local and galactic processes. Voyager 1's ongoing expedition affords a unique vantage point to observe these dynamics, shedding light on the interstellar medium's intricate structure and behavior.
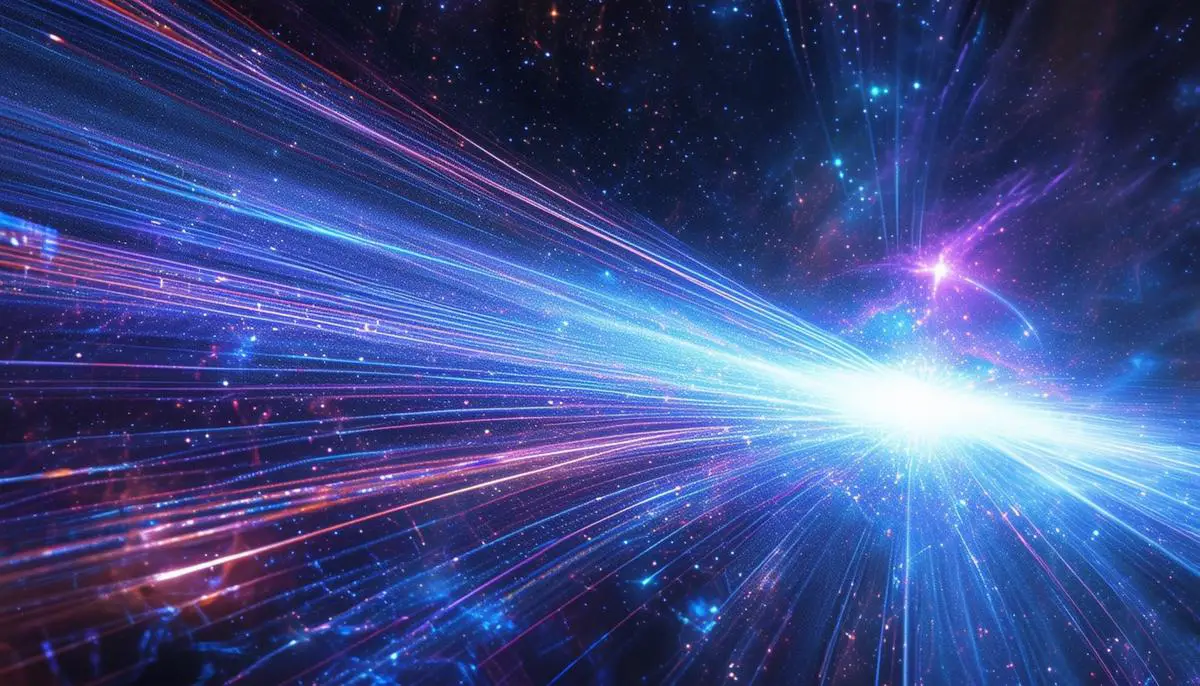
Scientific Instruments and Discoveries
Voyager 1 is equipped with a suite of scientific instruments designed to collect detailed information about the environments it traverses. Despite its journey into interstellar space, many of these instruments continue to function, providing unprecedented data far beyond the original mission's expectations. These instruments include:
- Plasma Spectrometer (PLS): While the PLS ceased functioning in 1980, data collected before its failure remain crucial. This instrument measured the properties of the solar wind, including its speed, density, temperature, and velocity distribution. The PLS data helped characterize the dynamic nature of the solar wind and its interactions with the heliosphere.
- Magnetometer (MAG): The MAG continues to measure the strength and direction of magnetic fields. Upon crossing the heliopause, the magnetometer detected the surprising continuity of the magnetic field's direction, challenging previous notions about abrupt changes expected at the heliosphere's boundary. This tool has been instrumental in studying the intricate magnetic interactions between solar and interstellar environments.
- Cosmic Ray Subsystem (CRS): This system measures high-energy particles originating from within and outside our heliosphere. One of the significant findings was the detection of a sharp increase in galactic cosmic rays upon crossing the heliopause. These observations revealed that the density and energy distribution of cosmic rays in interstellar space differ substantially from those within our solar shield, reshaping our understanding of cosmic ray propagation.
- Low-Energy Charged Particle Instrument (LECP): The LECP measures the energy and angular distributions of low-energy particles. Since crossing into interstellar space, it has detected variations in these particles, contributing to the understanding of how they interact with the interstellar medium and are affected by both local and galactic processes.
- Plasma Wave Subsystem (PWS): This instrument is critical for understanding the plasma environment Voyager 1 travels through. It detects the density of electrons in the space surrounding the spacecraft by measuring the quasi-thermal noise spectrum of plasma. In interstellar space, the PWS has recorded a significant increase in plasma density, providing direct evidence of the probe's entry into this new region and offering insights into the properties of interstellar plasma.4
One of Voyager 1's most notable scientific achievements since entering interstellar space is the revelation of the heliopause's porous nature. Voyager's instruments have shown that particles from the heliosphere can leak into interstellar space, contradicting earlier models that assumed a more distinct and impermeable boundary. This discovery suggests that interactions at the heliopause are far more intricate, involving continuous particle exchange between the solar and interstellar environments.
The continuous monitoring of plasma density has underscored the dynamic nature of the interstellar medium. Understanding how the local interstellar environment responds to solar activities, such as coronal mass ejections, elucidates how interstellar space is influenced by intra-solar events even at vast distances.
Voyager 1's ability to communicate detailed magnetic field data has also revolutionized our grasp of cosmic magnetic structures. The unexpected alignment of the interstellar magnetic field with that of the solar wind suggests potential reconnection processes or interactions previously unconsidered, laying the groundwork for new theories on how these fields overlap and influence one another.
Future Projections and Milestones
Voyager 1's future journey is replete with fascinating milestones as it ventures further into the interstellar domain. Once it exhausts its power supply, the spacecraft will continue its silent voyage, driven solely by inertia and the gravitational influences it encounters.
In the nearer term, Voyager 1 is set to pass through the Oort Cloud, a vast and distant region of icy bodies surrounding the solar system. This journey will begin around 300 years from now when the probe reaches the inner boundary of the Oort Cloud. This enormous sphere is thought to contain icy entities left over from the formation of the solar system, and crossing it will be a monumental chapter in Voyager 1's saga. The spacecraft will spend thousands of years navigating through this region, likely taking 30,000 years to exit completely.
After escaping the Oort Cloud, Voyager 1 will continue on its trajectory to the constellation Ophiuchus. The long-term projections indicate that the probe will approach its closest stellar encounter in approximately 40,000 years. This star, known as AC+79 3888, is located in the constellation Camelopardalis. While Voyager 1 will not come directly into the system, passing within 1.6 light-years is the closest it will get, which, while remote, is still significant given the vast scale of space.
The spacecraft's power longevity is another element of its remarkable journey. Voyager 1's plutonium-238 power source is expected to sustain one of its scientific instruments until approximately 2025. After this point, likely it will lose the capacity to power any of its instruments, ending its ability to collect and transmit scientific data. This means that we will no longer receive updates from the probe, marking the end of an era of unprecedented exploration. Nonetheless, though silent, Voyager 1 will persist on its trek as a herald from Earth, encapsulating humanity's quest for knowledge and our drive to explore the cosmos.
Post-communication, Voyager 1 will drift through interstellar space indefinitely unless it encounters significant gravitational disruption or collisions. The empty regions between stars provide a relatively undisturbed path, suggesting Voyager 1 could traverse the galaxy for millions – even billions – of years. Its destination remains unknown, but its pioneering spirit will endure, potentially bearing witness to stellar evolutions and galactic events far beyond our current horizon of comprehension.
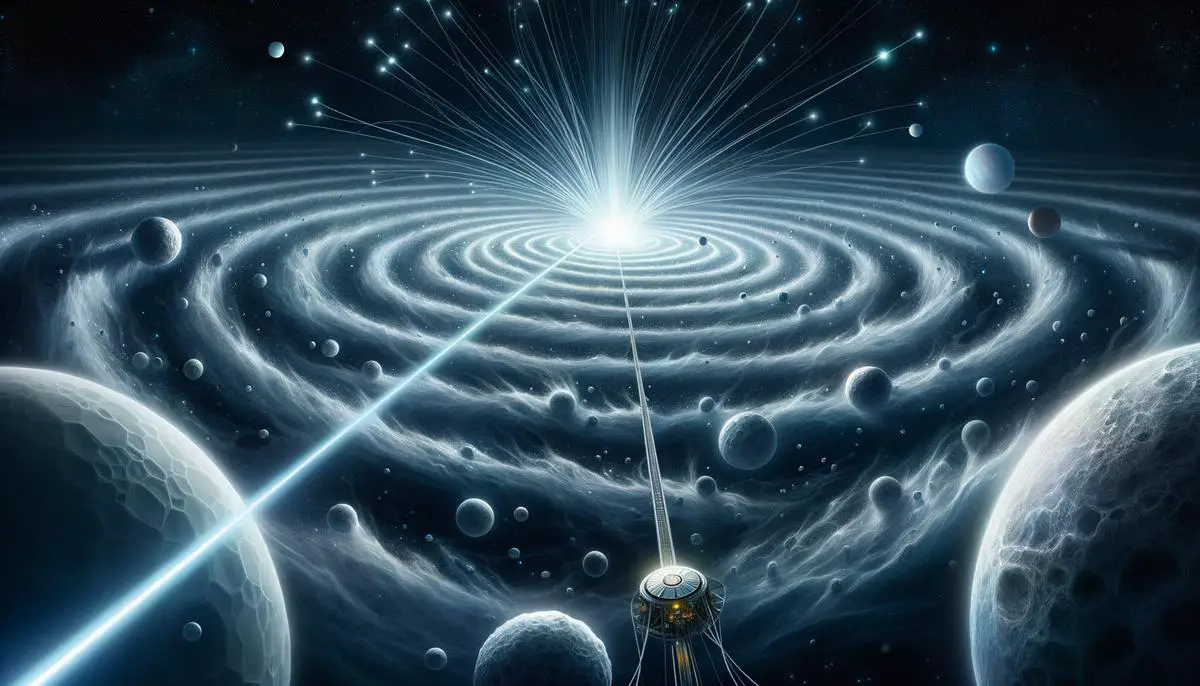
- Stone EC, Cummings AC, McDonald FB, Heikkila BC, Lal N, Webber WR. Voyager 1 observes low-energy galactic cosmic rays in a region depleted of heliospheric ions. Science. 2013;341(6142):150-153.
- Morabito LA, Synnott SP, Kupferman PN, Collins SA. Discovery of currently active extraterrestrial volcanism. Science. 1979;204(4396):972.
- Gurnett DA, Kurth WS, Burlaga LF, Ness NF. In situ observations of interstellar plasma with Voyager 1. Science. 2013;341(6153):1489-1492.
- Gurnett DA, Kurth WS, Stone EC, et al. Precursor and termination shock particles in the heliosheath. Astrophys J. 2015;809(2):121.