Observable Universe and Its Scale
The observable universe spans an awe-inspiring 93 billion light-years, equivalent to about 8.8 × 10^23 kilometers. Within this vast expanse lies approximately 3.1 × 10^54 kilograms of matter, with an average density of just a few atoms per cubic meter.
Astronomers employ unique units to measure cosmic distances:
- Parsec (pc): 3.1 × 10^13 kilometers
- Kiloparsec (kpc): 1000 parsecs
- Megaparsec (Mpc): 1,000,000 parsecs
The majority of the universe is empty space, with matter organized into various formations. The elemental composition of the observable universe is predominantly:
- Hydrogen: 75% of baryonic mass
- Helium: 23%
- Heavier elements (metals): 2%
Stars, the cosmic furnaces, undergo nuclear fusion, shining for billions of years until they exhaust their fuel. Upon depletion, stars transform into white dwarfs, neutron stars, or black holes, depending on their initial mass.
Galaxies exist in three main types:
- Spirals (like our Milky Way)
- Ellipticals
- Irregulars
The Milky Way, a spiral galaxy, stretches about 30 kiloparsecs in diameter. Dark matter, though invisible, plays a crucial role in influencing galactic structure.
On the grandest scale, galaxies group into clusters and superclusters, connected by filaments in a cosmic web. This intricate structure provides valuable insights into the nature of dark matter and dark energy, driving ongoing research in cosmology and astrophysics.
Baryonic Matter and Stellar Evolution
Baryonic matter, the stuff of everyday life, consists primarily of protons, neutrons, and electrons. Stars exemplify the dynamic life cycles of this matter in the cosmos.
The stellar life cycle begins with the gravitational collapse of gas and dust clouds. When conditions are right, nuclear fusion ignites, marking the birth of a star. At its core, hydrogen fuses into helium, releasing energy that maintains hydrostatic equilibrium against gravitational forces.
A star's mass dictates its destiny:
- Massive stars: Burn fuel rapidly, shining brightly for millions of years
- Low-mass stars (like our Sun): Burn slowly, lasting billions of years
As nuclear fuel depletes, stars undergo dramatic transformations:
- Sun-like stars expand into red giants
- Outer layers are shed, forming planetary nebulae
- Core contracts to become a white dwarf
Massive stars face a more explosive fate:
- Supernova explosion
- Formation of neutron stars or black holes
These stellar lifecycles illustrate the universe's cyclical nature: birth through fusion, life via nuclear burning, and death into remnants. Each phase offers profound insights into celestial mechanics and our cosmic heritage.

Galaxies and Their Types
Galaxies, vast assemblies of stars and cosmic dust, are categorized into three primary types:
- Spiral galaxies: Like our Milky Way, featuring:
- Flat disks with spiral arms
- Young, hot stars marking areas of stellar birth
- Central bulge of older stars
- Elliptical galaxies:
- Spherical or elongated structures
- Populated by old, cool stars
- Little to no active star formation
- Irregular galaxies:
- No definitive structure
- Often result from cosmic mergers or near misses
- Feature chaotic regions of intense star formation
Dark matter, though invisible, plays a crucial role in shaping galactic structures through its gravitational influence. It acts as a cosmic scaffold upon which galaxies grow and evolve.
Galaxies are social entities, gathering in clusters bound by mutual gravitational attraction. These clusters, in turn, form even larger assemblages called superclusters. Studying these immense structures provides valuable insights into the universe's large-scale operations and the profound influence of dark matter and dark energy.

Cosmic Web and Large-Scale Structures
The cosmic web forms the universe's large-scale structure, consisting of:
- Vast filaments
- Expansive voids
- Dense galaxy clusters
This intricate network offers crucial insights into the nature of dark matter and dark energy.
Filaments: These cosmic highways stretch tens of millions of light-years, connecting galaxy clusters. Their higher material density compared to surrounding voids indicates the presence of dark matter.
Voids: Regions with fewer galaxies, emphasizing the distributed nature of dark matter and delineating the boundaries of the cosmic web.
Galaxy clusters: Dense knots of tumultuous activity, formed over billions of years from early density fluctuations. They demonstrate gravitational evolution and the binding role of dark matter.
Dark energy drives the universe's expansion, subtly influencing the separation of galaxies. The cosmic web serves as both a map and barometer of these forces, each structure silently narrating the enigma of dark energy.
Scientists employ advanced tools to study these formations:
- Redshift mapping
- Radio observations
These techniques help refine our understanding of dark matter and dark energy's roles in cosmic evolution, pushing the boundaries of our knowledge about the universe's fundamental nature.
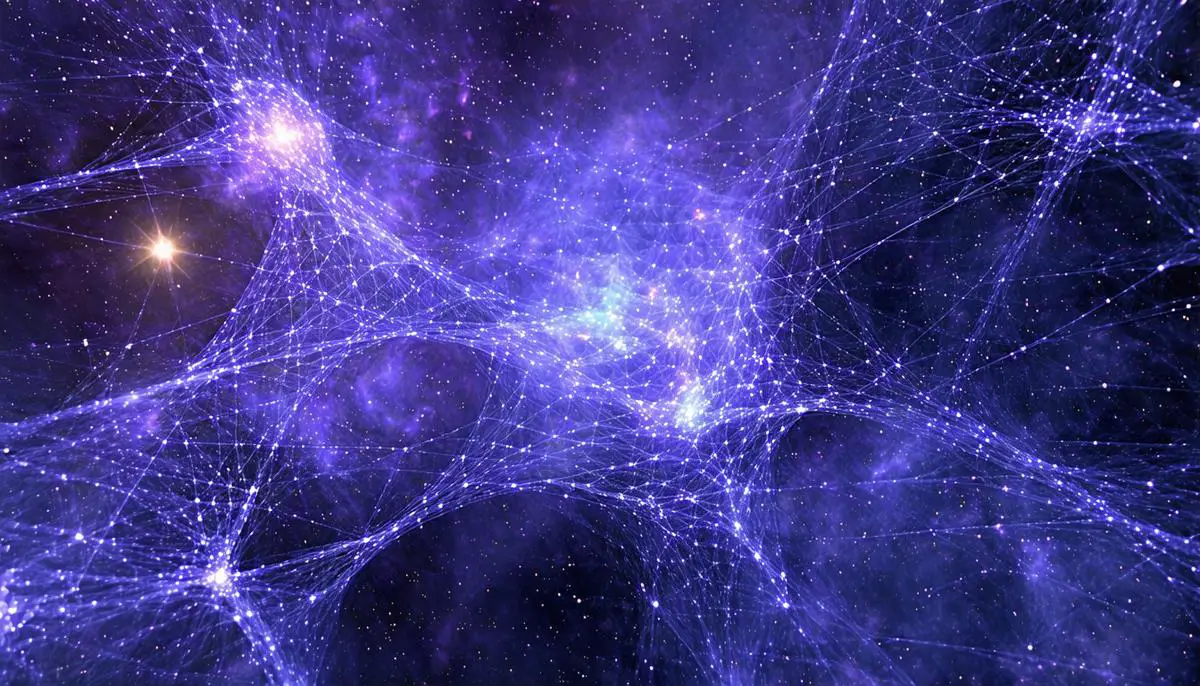
Cosmological Principles and Anomalies
The Cosmological Principle asserts that the universe is homogeneous and isotropic on the largest scale. This foundational concept shapes our understanding of the cosmos and forms the basis for models like the Big Bang theory.
Weak gravitational lensing allows astrophysicists to examine the distribution of matter throughout the universe. This technique helps verify adherence to the Cosmological Principle or identify potential anomalies.
"Measuring the dipole is an extremely important test of cosmology, and can tell us whether our fundamental assumptions about the structure of the Universe are correct." – Jonah Wagenveld, MPIfR astronomer
Questioning isotropy could lead to profound implications for our understanding of cosmic order. Large-scale anisotropies might necessitate rethinking core cosmological models, including:
- The universe's geometry
- Expansion history
- Fundamental physics
The Euclid space telescope aims to map billions of galaxies, potentially uncovering subtle deviations from universal homogeneity. Discrepancies in measurements of the universe's expansion rate hint at underlying physics beyond contemporary models.
The interplay of dark matter and dark energy influences large-scale dynamics and may affect observed irregularities. Studying these forces offers a pathway to deeper cosmic comprehension.
As we continue to probe the universe's vastness, potential anomalies challenge us to revisit and refine our understanding of the cosmos. Each discovery invites us to learn and potentially redefine what we know about our infinite universe.

As we explore the universe, we uncover the intricate interplay of cosmic forces and structures that shape our understanding. Each discovery and anomaly adds to our knowledge, encouraging deeper investigation. In this grand structure, the pursuit of knowledge becomes a journey of wonder and revelation.
- Valade A, Libeskind NI, Pomarède D, et al. Identification of basins of attraction in the local Universe. Nat Astron. 2024.
- Adam J, et al. Testing isotropy using weak gravitational lensing with Euclid. J Cosmol Astropart Phys. 2025.
- Gupta N, et al. The MeerKAT Absorption Line Survey (MALS): First results. Astron Astrophys. 2024.
- Wagenveld J, et al. Measuring the cosmic radio dipole with MeerKAT. Astron Astrophys. 2024.