The story of the Hubble Constant reflects humanity's pursuit of understanding the universe's expansion, marked by significant milestones and ongoing debates.
Historical Development of the Hubble Constant
Edwin Hubble's observation that galaxies are moving away from us revolutionized our cosmic perspective. Before Hubble, Vesto Slipher noted that spiral nebulae were racing through space, providing the first observational evidence of an expanding universe.
Initially, Hubble estimated the expansion rate at 500 kilometers per second per megaparsec, an overestimation that required refinement. Cepheid variables, stars with pulsating brightness, helped astronomers map the universe's structure more accurately.
By mid-20th century, advances in technology, including radio telescopes, led to more reasonable estimates. The understanding of type Ia supernovae as standard candles also improved cosmic distance measurements.
The Hubble Space Telescope's launch in 1990 brought a significant leap forward, allowing scientists to peer deeper into the cosmos and refine the Hubble Constant with greater precision.
Despite progress, debates about the exact value persist, leading to the "Hubble Tension." Different measurement methods suggest slightly different expansion rates, potentially implying new physics or undiscovered aspects of current models.
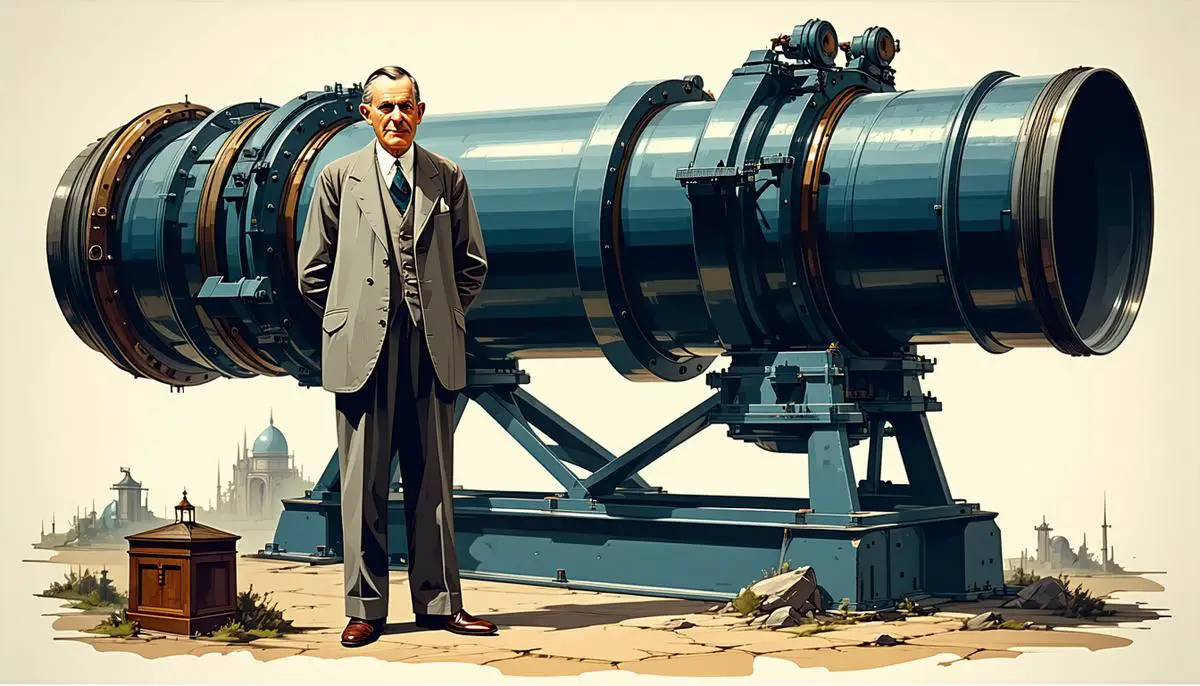
Methods of Measuring the Hubble Constant
Astronomers have developed various methods to determine the Hubble Constant, each with its own challenges:
- Cosmic distance ladder: A step-by-step approach using nearby objects like Cepheid variables and extending outward using type Ia supernovae as standard candles.
- Gravitational lensing: Studying the time delay between light paths of multiple images created by massive objects to infer distances and the Hubble Constant.
- Cosmic microwave background (CMB): Analyzing primordial ripples in the early universe snapshot to determine the expansion rate.
Each method has its uncertainties, driving cosmologists to refine techniques and improve precision through collaboration and advanced technology. The ongoing debate surrounding the Hubble Constant reflects the dynamic nature of astrophysical research and our enduring quest to understand the universe.
The Hubble Tension
The Hubble Tension arises from discrepancies between different methodologies used to evaluate the universe's expansion rate. Local cosmic methods, using Cepheid variables and type Ia supernovae, typically yield a higher Hubble Constant than measurements probing the early universe through the cosmic microwave background (CMB).
This discrepancy has profound implications, potentially indicating flaws in our cosmic model or hinting at undiscovered phenomena such as exotic forms of dark matter or energy. The tension doesn't signal defeat but rather invigorates scientific inquiry, prompting new theories and technologies to bridge the divide.
Scientists remain committed to resolving this tension, whether by refining the standard model or exploring beyond established paradigms. The ongoing pursuit of clarity continues to inspire and drive our understanding of the expanding universe.

Role of Modern Telescopes in Refining the Hubble Constant
Modern telescopes play a crucial role in refining our understanding of the Hubble Constant:
- James Webb Space Telescope (JWST): Captures celestial phenomena with unprecedented sharpness in the infrared spectrum, allowing for more precise observations of Cepheid variables and type Ia supernovae.
- Planck spacecraft: Mapped the cosmic microwave background with extraordinary accuracy, offering a precise snapshot of primordial fluctuations.
These advanced instruments provide evidence that prompts thorough examination of our cosmological models. Their detailed observations help bridge the Hubble Tension, encouraging astronomers to consider novel solutions and engage in creative theorizing.
While the Hubble Tension persists, the contributions of these modern telescopes light the way toward a more coherent cosmic narrative, turning uncertainties into opportunities for discovery.
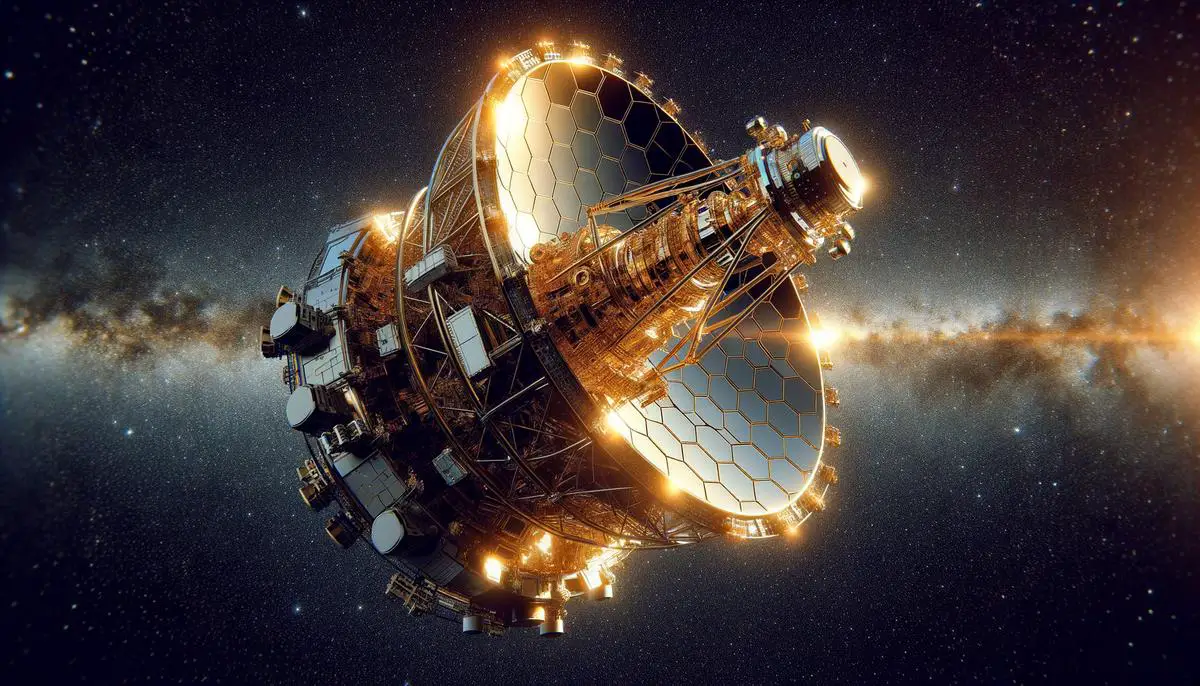
Future Directions in Hubble Constant Research
Future research projects and technologies promise to shed light on the Hubble Tension and refine the Hubble Constant:
- Dark Energy Spectroscopic Instrument (DESI): Aims to map the redshifts of millions of galaxies, potentially offering a more refined value for the Hubble Constant.
- European Space Agency's Euclid mission: Will survey billions of galaxies, deepening our understanding of dark energy, dark matter, and cosmic inflation.
- Vera C. Rubin Observatory: Will enable detailed observations of transient celestial events, refining critical distance measurements.
- NASA's Nancy Grace Roman Space Telescope: Expected to provide pivotal insights for cosmic distance measures and exploring the forces propelling the universe's expansion.
These initiatives offer optimism in resolving the Hubble Tension, each contributing to our understanding of the cosmos. Whether through revising cosmological frameworks or revealing new phenomena, these endeavors propel us toward potentially groundbreaking discoveries, inspiring continued exploration of our vast universe.
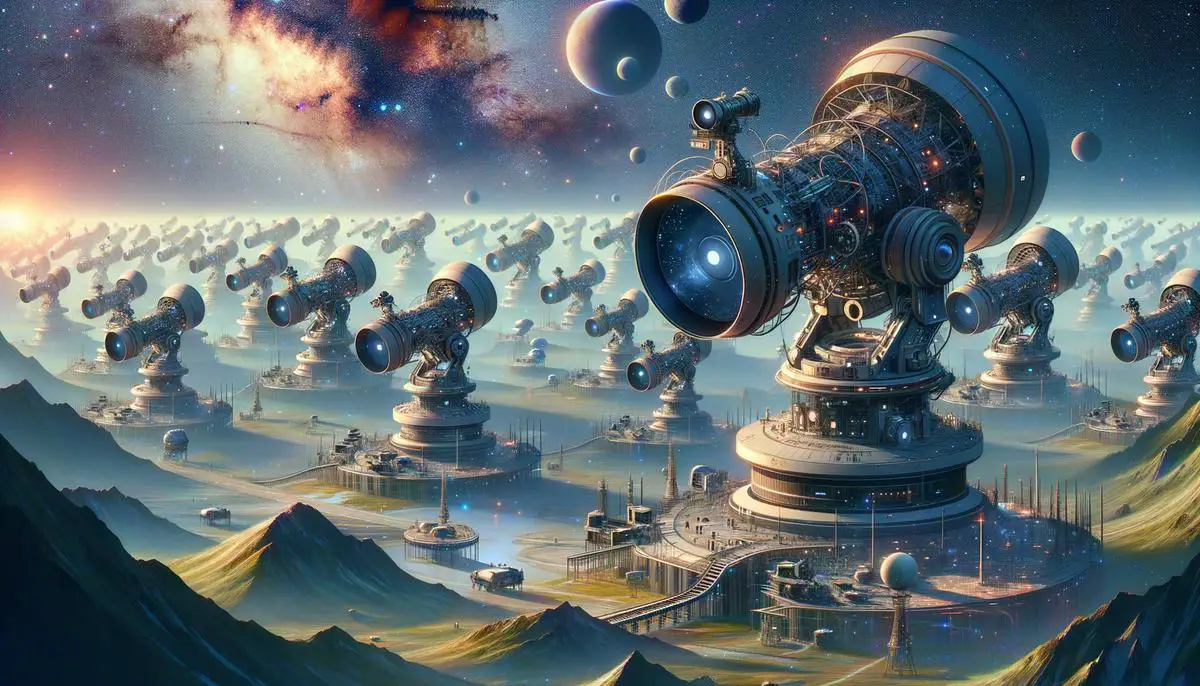
The ongoing quest to refine the Hubble Constant enhances our comprehension of universal expansion and inspires further exploration of the cosmos.
- Freedman WL, Madore BF. The Hubble Constant. Annu Rev Astron Astrophys. 2010;48:673-710.
- Riess AG, Macri LM, Hoffmann SL, et al. A 2.4% Determination of the Local Value of the Hubble Constant. Astrophys J. 2016;826(1):56.
- Planck Collaboration, Aghanim N, Akrami Y, et al. Planck 2018 results. VI. Cosmological parameters. Astron Astrophys. 2020;641:A6.
- Verde L, Treu T, Riess AG. Tensions between the early and late Universe. Nat Astron. 2019;3:891-895.
- Scolnic D, Riess AG, Huber ME, et al. The Complete Light-curve Sample of Spectroscopically Confirmed SNe Ia from Pan-STARRS1 and Cosmological Constraints from the Combined Pantheon Sample. Astrophys J. 2018;859(2):101.