Through the vast stretches of space, unique celestial bodies spin, tilt, and orbit in a fascinating galactic ballet. Our focus here is to unravel the complexities that go into determining the day length of planets. We are going to delve deep into how planetary rotation, influenced by factors like rotation speed and equatorial bulge, directly impacts day length. Furthermore, the importance of eccentricity of a planet’s orbit and its effect on day length, be it a perfect circle or an extreme ellipse, will be explored. We also examine the impact of axial tilt on day lengths across different seasons. The subtle yet significant influences of atmospheric refraction and gravity-tidal interactions in determining the length of a day on a planet complete the investigation into this intricate celestial phenomenon.
Planetary rotation and its connection to day length
Title : The Phenomenon of Planetary Rotation and Day Length
In the grand and infinitely expansive theater of the cosmos, countless dramas of celestial bodies ensue on a scale that leaves one humbled. Among these celestial bodies, planets, especially those in our solar system, offer an intriguing example of the harmony and precision exhibited by nature. A noteworthy aspect that governs a significant part of life on these planets, notably Earth, is the planetary rotation and its impact on the length of a day.
Planetary rotation can be conceptualized as a self-spin that a planet performs on its axis. Imagine observing a spinning top from above; the motion it exhibits will give a first-hand understanding of how a planet rotates.
A crucial aspect of this rotation is the duration it takes for one complete spin. This duration unequivocally determines the length of a day on the respective planet. On our home planet Earth, this rotation takes approximately 24 hours, which is universally accepted as one day. This explains why our days and nights are equally divided into 12 hours each.
Another prominent example within our solar system is Mars, our next-door neighbor. Mars takes slightly longer than Earth to complete its rotation, specifically about 24 hours and 40 minutes. Consequently, a Martian day, or sol, is longer than an Earth day.
Contrastingly, the gas giant Jupiter, with its colossal mass, completes a super-speed rotation in just about 9 hours and 56 minutes, making a Jovian day remarkably shorter.
Venus, however, presents an intriguing case. It takes a whopping 243 Earth days to complete one rotation, making it unique as the planet with the longest day in the solar system. This languid rotation results in its day being longer than its year, which takes only 225 Earth days!
One point to be considered is how the rotational speed affects the perceived daylight on the planet’s surface. A slower rotation would mean longer periods of exposure to the sun, resulting in longer days. Conversely, quicker rotations lead to more frequent alternation between daylight and darkness, hence shorter days.
However, it’s pertinent to note that rotation alone doesn’t dictate day length exclusively. The planet’s axial tilt and orbital shape around its star also play significant roles. These factors combine to create various patterns of daylight and darkness, further influencing the planet’s climatic and geological aspects.
In conclusion, while it may seem like a mundane reality to us earthlings, the occurrence of day and night is a fascinating scientific phenomenon determined primarily by our planet’s rotation. Not only does it impact daily routines and biocycles, but it also determines the larger geological and climatic patterns of the planet, further reflecting the intricate and harmonious mechanics of the cosmic ballet.
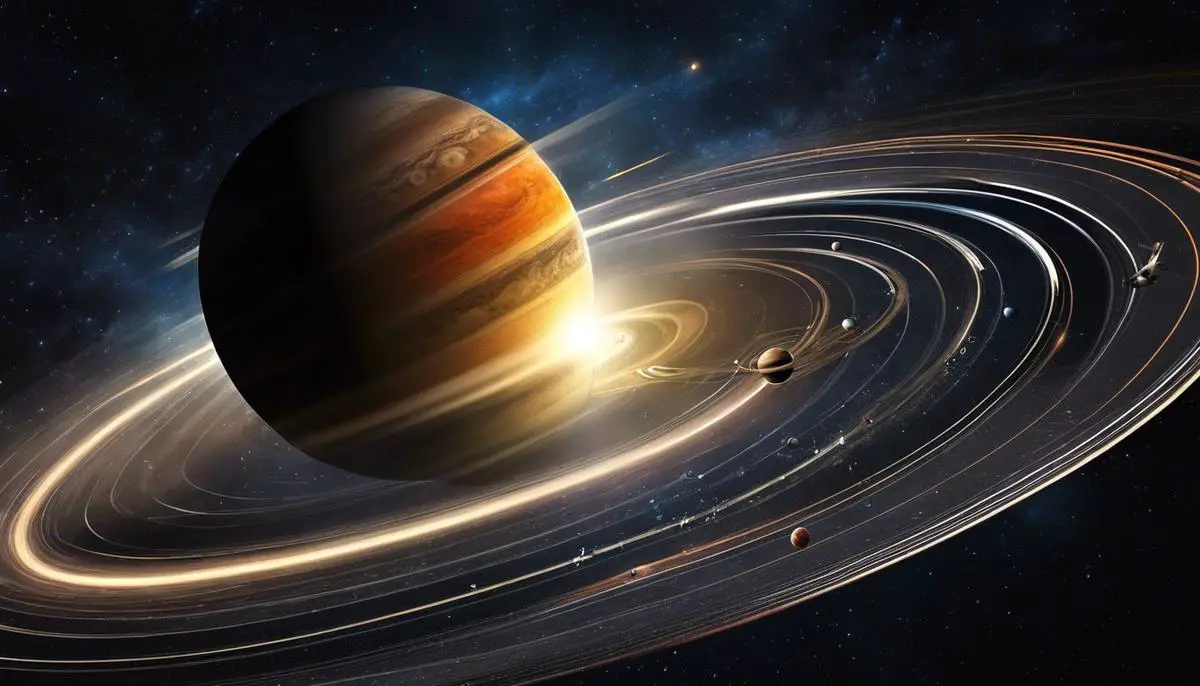
The influence of orbital eccentricity on day length
The Influence of Orbital Eccentricity on Day Length: Expanding the Planetary Science Paradigm
Delving further into the field of planetary science, the eccentricity of a planet’s orbit, the measure of how much it deviates from a perfect circle, bears significant relevance to the topic under discussion – the length of a day. The understanding of this factor bursts forth from traditional paradigms clustering around axial tilt and rotational speed, carving out a niche for itself in the complex mechanics that govern planetary day length.
The term “eccentricity” might cast images of confounding complexity, but consider it as follows: Imagine a stretched rubber band which, when let go, snaps back into its original round form. This is analogous to a planet’s orbit; gravity eternally tugs at it, bidding it to curve around a central body like our Sun. In an ideal scenario, this would result in an orbit that’s perfectly circular. However, in reality, most orbits, including Earth’s, bear more resemblance to an ellipse or an oval. This deviation from the “perfect circle” is what is referred to as orbital eccentricity.
Before proceeding further, it is important to clarify a common misconception. A planet’s orbital eccentricity does not directly affect the length of a day — rather, it influences phenomena that subsequently impact day length. Eccentricity’s role is intricately linked with axial tilt and rotational speed, factors previously discussed, creating a cause-and-effect chain that ultimately bears impact on the length of a planetary day.
As the eccentricity of a planet’s orbit increases, it spends different amounts of time at various points in its orbit. Due to Kepler’s second law of planetary motion, the planet moves faster when closer to the Sun (perihelion) and slower when farther away (aphelion). This variation in speed instigates an effect known as “equation of time”, which results in apparent solar time deviating from mean solar time. In layman’s terms, this means the position of the Sun in the sky, as observed from the planet’s surface, does not exactly match the time displayed on our clocks.
For instance, if we benchmark the Earth’s rotational period at perihelion and compare it with the period at aphelion, a discrepancy arises. What we perceive as a 24-hour day is actually a “mean solar day,” an average over the course of Earth’s elliptical orbit. Yet, the eccentricity of Earth’s orbit is relatively low, making this effect perceivable, albeit minimal.
On planets with high eccentricity like Mercury, these effects are profoundly exaggerated. Mercury’s highly eccentric orbit combined with its gravitational interactions with the Sun has led to a peculiar phenomenon. Mercury spins three times on its axis for every two orbits around the Sun, resulting in the ‘Mercurian day’ (one solar day from sunrise to the next) being longer than its year.
Hence, unpacking the biomechanical intricacies, the influence of a planet’s orbital eccentricity on day length is indirect, but far from insignificant. This subtle interplay is an exemplar of the vast cosmic logic that binds us, bridging the gap between the astronomical and the terrestrial, the cosmic and the quotidian. A synecdoche of planetary science, it demonstrates that even the most seemingly esoteric celestial mechanics bear profound implications – not only for a planet’s climatic patterns and geological changes, but also its potential to host life.
Thus, studying orbital eccentricity is not merely an exercise in academics but also a quest for profound understanding that transcends the microcosm of Earth, eventually opening avenues for interstellar exploration and extraterrestrial discovery. Indeed, the allure of discovering the unknown and unearthing truths about our universe enamors every eager mind, reinforcing the role of scientific inquiry in unlocking the secrets of the cosmos.
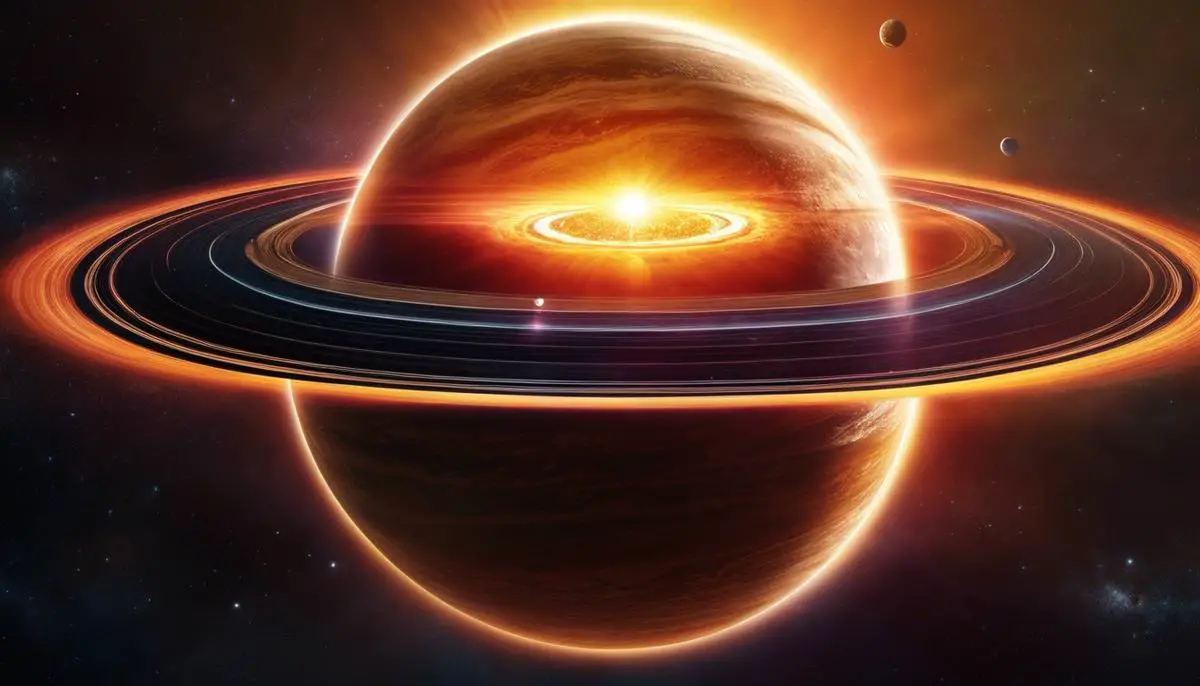
Effects of axial tilt on day lengths
Building on the concept of axial tilt and its influence on the planetary day length, it is important to expound on how the tilt affects the distribution of sunlight on a planet’s surface, thereby influencing day length.
The axis of a planet, the imaginary line through its North and South poles, can lean towards or away from its orbit around the sun. This lean or ‘axial tilt’ can greatly affect the planet’s climate and, in turn, the length of its days.
Different planets have varying degrees of axial tilt. Earth, for instance, has an axial tilt of about 23.5 degrees. This tilt is what causes our four distinct seasons and affects our day length. When the North Pole leans towards the sun, we experience summer in the Northern Hemisphere. Because of the tilt, the sun can stay above the horizon for longer, thus giving us longer days.
On the other hand, Uranus, the seventh planet from the sun, has an extreme tilt of about 98 degrees. Instead of spinning upright, it essentially rolls around the sun on its side. This severe tilt results in extreme seasonal changes, with each pole experiencing 42 years of continuous daylight, followed by 42 years of darkness. The drastic changes in sunlight distribution significantly impact the planet’s day length.
Conversely, a planet like Mercury, with virtually no tilt (just 0.03 degrees), has minimal variation in day length. Complicating matters, though, Mercury’s day is influenced more by its highly eccentric orbit and slow rotation. Here, axial tilt plays a minor role in affecting day length.
The subtleties in how axial tilt affect day length may seem complex, but they are critical to understanding planetary climates and the potential for life on other planets. Researchers in this field work tirelessly to decipher these celestial mechanics. There’s a sense of beauty in the predictive patterns they reveal, a balletic dance where each celestial body follows its own rhythmic steps.
How intense or mild the seasons are, how long daylight persists, these are all metronomic ticks that signal a planet’s axial tilt and its effect on day length. The formula for determining day length is not mired solely in rotation speed or orbital eccentricity, but a symphony of factors that include axial tilt among its key notes.
Understanding these planetary dynamics is a complex study, bound in equations and models. However, at its core, it is driven by a curiosity to understand the cosmos and our place within it. These investigations deepen our grasp of the universe, nurture our appreciation for Earth’s unique balance, and spur future explorations. They highlight the intricate beauty of cosmic mathematics and foster profound reflections on what other wonders the universe has yet to reveal.
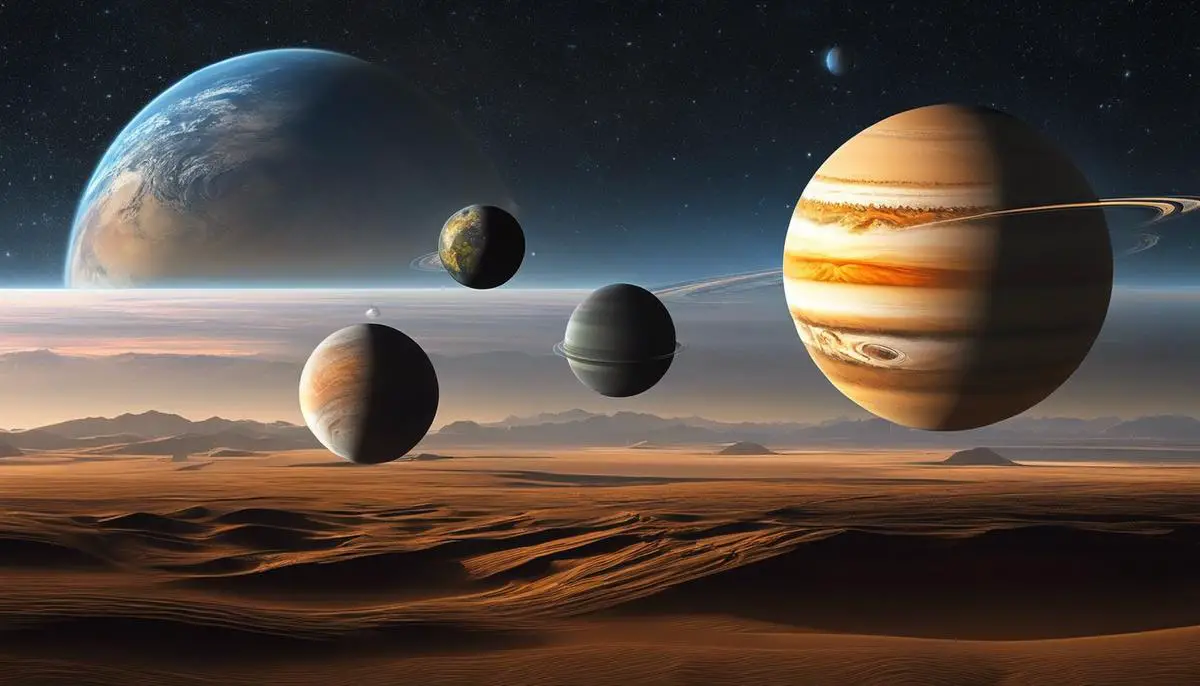
Atmospheric refraction and day length
Stepping on:
Atmospheric refraction now comes under the microscope. Primarily, atmospheric refraction is caused by the varying density of Earth’s atmosphere. When the sun is directly overhead, sunlight travels through the least possible amount of the Earth’s atmosphere, meeting minimal resistance, and hence, minimal refraction. However, during sunrise and sunset, sunlight traverses a larger cross-section of the Earth’s atmosphere, and due to the differing densities it encounters, refraction occurs.
This bending, or “refraction,” of light rays alters our perception of where the sun is in the sky. Thus, the phenomena of sunrise and sunset are visible slightly earlier and later than if the Earth had no atmosphere. This phenomenon effectively lengthens our perception of daylight, even if the astronomical definition of day – the period during which a celestial body makes one full rotation on its axis – remains unchanged.
Although this effect is subtle, accounting for roughly 6 to 7 minutes of additional daylight on Earth, understanding it is crucial for accurate timekeeping. Civil time, as most of us use, is based on mean solar time, taking into account not just the planet’s rotation but also the impact of atmospheric refraction on the apparent position of the sun.
Juxtaposing to other bodies in our solar system, the influence of atmospheric refraction on perceived day length would vary. For instance, on Mars, which has a thinner atmosphere than Earth’s, the refractive lengthening of the day would likely be less. Contrarily, on a planet like Venus, cloaked in a dense and thick atmosphere, the effect of atmospheric refraction might be significantly more pronounced.
Exploring these nuances underscores that day length generates from a symphony of factors playing together harmoniously – planetary rotation, axial tilt, orbital shape, and certainly, atmospheric refraction. And as with any harmonious entity, it is the intricate interactions, even those seemingly minor like refraction, that cumulatively make the whole.
In conclusion, observations and understanding of our own Earth’s system serve as an essential point of comparison for characterizing other seemingly alien environments. Moreover, it brings to focus the magnificent symphony of physics and cosmic forces that are constantly at play in the universe we inhabit. A cosmos that, albeit its unfathomable size, obeys a set of intricate and beautiful rules that allow the clockwork precision of celestial bodies such as the very planet we call home.
The profound knowledge that emerges extends beyond academia, fostering greater appreciation for Earth’s life-sustaining balance and inspiring future explorations.
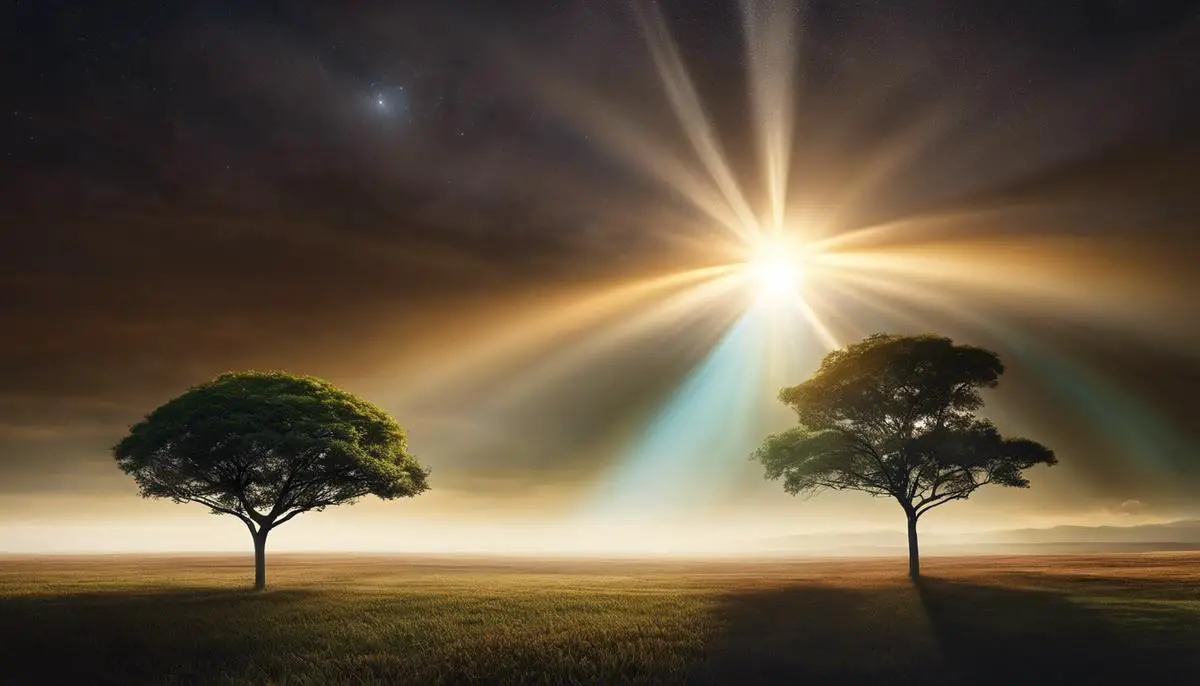
Gravity and tidal interactions and their influence on day length
So, after diving deeply into the complex mechanisms determining day length on the celestial bodies, we grasp a more refined understanding of the universe in which we live. The intricate interplay of planetary rotation, orbital eccentricity, axial tilt, and atmospheric refraction all meticulously contribute to the uniqueness of each planet’s day length. It is the presence of these variations that makes each planet, including our own, an entity with its own unique rhythmic cycle. The hidden influence of gravity and tidal interactions further emphasizes the complexity and interdependence within our vast cosmic sea. Understanding these phenomena underscores the meticulous precision of the laws of physics that govern not just our own world but the cosmic stage as a whole.