Defining the Speed of Light
The speed of light, measured as approximately 299,792 kilometers per second, stands as a cornerstone of physics and a universal speed limit according to the laws of our Universe. This concept was first quantitatively suggested by Ole Roemer in 1676, who deduced that light must have a finite speed by observing the moons of Jupiter.
In the 19th century, James Clerk Maxwell inferred that light was an electromagnetic wave, traveling through the vacuum of space at a constant speed. He calculated this speed using the known electromagnetic constants at the time, remarkably arriving at numbers close to those measured by astronomers and physicists today.
The precise figure was refined through multiple innovative experiments, including those conducted by Albert A. Michelson. Using a device known as the interferometer, Michelson was able to make unprecedentedly accurate measurements of the speed of light that approached today's accepted values.
In modern settings, this measurement virtually all areas of physics and binds our understanding of the universe's space-time fabric. Causality itself—the concept that cause precedes effect—is grounded by light's consistent speed, as postulated in Einstein's theory of relativity.
The importance of understanding light's constant speed influences everything from satellite communication and GPS systems to fundamental questions about the origins and fate of the universe. Each careful measurement and refined theory unfolds yet another layer in our grasp of light and the very fabric binding the universe.
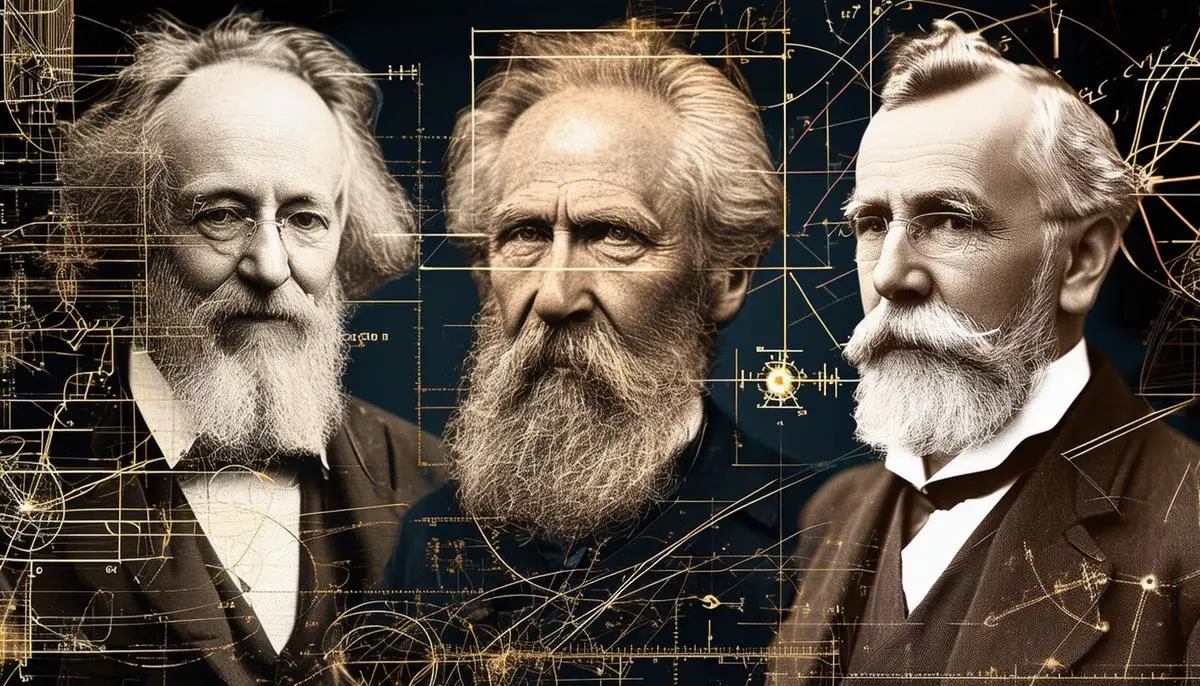
Physical Boundaries Set by Light Speed
From experiments and detailed deductions, scientists have embraced the startling reality that nothing with mass can surpass or even meet the speed of light. According to Einstein's theory of relativity, as an object with mass accelerates towards the speed of light, its relativistic mass increases, requiring ever-greater amounts of energy for continued acceleration. Attaining the speed of light would necessitate infinite energy, a condition that is physically unattainable.
Consequently, the speed of light places a hard cap on how fast any material object can travel. This universal speed limit is more than just a threshold that cannot be crossed; it is a central principle that many fundamental concepts in physics.
Achieving Near-Light Speeds
Modern technological efforts to approach the speed of light embody the blend of theoretical physics and ambitious engineering. While reaching or surpassing the speed of light remains impossible for objects with mass, current advancements focus on attaining speeds that fractionally near this cosmic speed limit.
One such proposal includes nuclear thermal propulsion, which attempts to harness the energy produced by nuclear reactions as a means of propulsion. Enhanced materials and novel reactor designs strive to safely achieve higher velocities that would potentially shorten interplanetary travel significantly.
Photon propulsion steps even deeper into theoretical territory but promises even greater speeds. This futuristic mechanism aims to utilize the momentum of photons—light particles—which, despite carrying no mass, exert a perceptible force when traveling in beams concentrated enough to propel spacecraft.
Behind these intriguing propulsion methods lie numerous challenges:
- The engineering complexities of constructing and maintaining mechanisms that operate under extreme conditions are formidable.
- The energy requirements are staggeringly high; accelerating even a small probe to near-light speeds demands amounts of energy that rival that of entire nations' capabilities.
- Even if a mission managed to reach these extraordinary speeds, managing communications and navigating at velocities close to light speed present unique challenges. At such rapid paces, traditional systems for guiding and synchronizing with a spacecraft would need rethinking.
The aspirations to bridge vast cosmic distances more quickly are driven by a profound yearning to explore, to reach beyond our current boundaries, and to understand more intimately the galactic expanse that surrounds us. While constrained by current technology, our progressive grasp over physics and propulsion continues to seed these ambitious goals, promising a future where our reach may gunnily skirm the light fantastic edges of space.
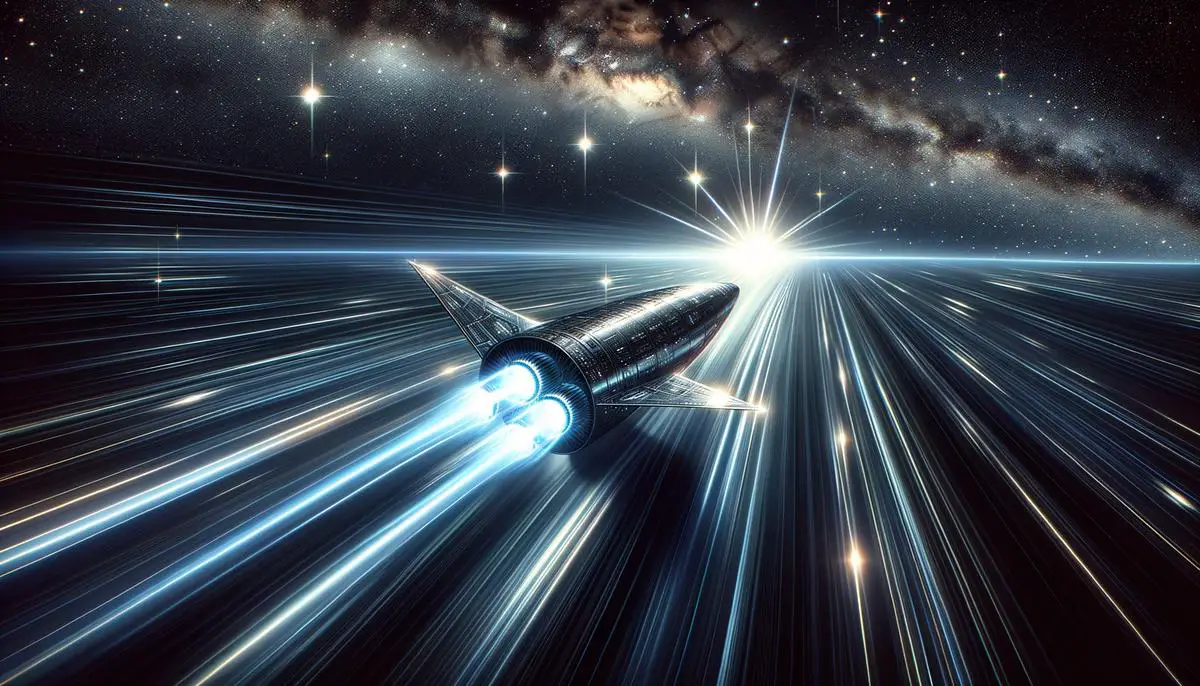
Light Speed in Different Mediums
As light waves travel from one medium to another—such as from air into water or glass—their speed changes, a phenomenon that intrigues physicists and plays a crucial role in technologies like fiber optics and laser communication. This remarkable behavior is primarily due to a property of the material called the refractive index, a crucial dimensionless figure that indicates how much a medium can bend light.
In a vacuum, light travels at its maximum speed of approximately 299,792 kilometers per second. When light penetrates materials like water or glass, it slows down measurably. The specific slowdown depends on the medium's refractive index: the higher the index, the slower light travels through that material.
The underlying mechanism for this behavior involves the interaction of light photons with the atoms within a medium. As photons enter a material, they engage with, and are absorbed by the atoms momentarily before being re-emitted in the same general direction but at a slightly delayed pace.
The bending of light, or refraction, associated with these speed changes, occurs because of the variation in light speed across different media. When light waves cross from a lower refractive index medium to a higher one, they slow down and bend towards the normal line that's perpendicular to the surface at the point of entry. Reverse happens when light switches from a high-refractive index medium to a lower one; it speeds up and bends away from the normal. This refractive property is pivotal in lenses to help us see clearly, focus cameras or microscopes, and even correct vision with eyeglasses.
Scientists and engineers manipulate light in various applications. One of the most common uses is in fiber-optic cables, where light signals carrying data adjust speeds as they whip through cables made of glass or plastic. These differing speeds and bending skills of light strands facilitate wide-range telecommunication and data transfer across the global internet infrastructure.
As we continue pioneering forward, the intricacies of how light behaves in different environments shine a beacon on greater, possibly revolutionary applications in both current and emerging fields of physics and technology.
Expanding Universe and Light Speed
As we ponder light speeding through the cosmos at an unvarying pace, the vast theatre of the universe expands. The fabric of the cosmos stretches, augmenting the distances between galaxies. This continuous expansion leads us into a counterintuitive realization: galaxies can appear to recede from each other at velocities surpassing the speed of light.
Consider a commonly used metaphor involving a balloon with dots marked on its surface. As the balloon inflates, the dots, which can represent galaxies, move further apart from each other. Notably, their movement isn't due to them propelling through the balloon's surface (space), but because the balloon's surface (the universe) is expanding.
In an expanding universe, light itself tells a fascinating story. The further away a galaxy is, the faster it seems to recede from us, thanks to the expanding universe. Thus, light from these distant galaxies traverses an ever-stretching fabric of space to reach us. As it travels, the wavelength of this light stretches too; a phenomenon observed as a redshift. Astronomers measure this redshift to infer how fast a galaxy moves away from us and consequently determine the expansion rate of the universe1.
This expansion and resultant speeds do not contravene general relativity or our understanding of causality and the constants of physics. The very fabric of space-time ensures light's speed remains a ceiling for local movement within space, not across space itself on cosmological scales.
This expounding of space introduces implications for observational astronomy and underscores our theorizations about the universe's destiny. As the universe expands, and assuming this expansion continues to accelerate, galaxies that are not gravitationally bound to us will eventually recede at such vast speeds that their light may never reach us—rendering them not merely distant, but invisible isolated islands in a swiftly stretching cosmic ocean.
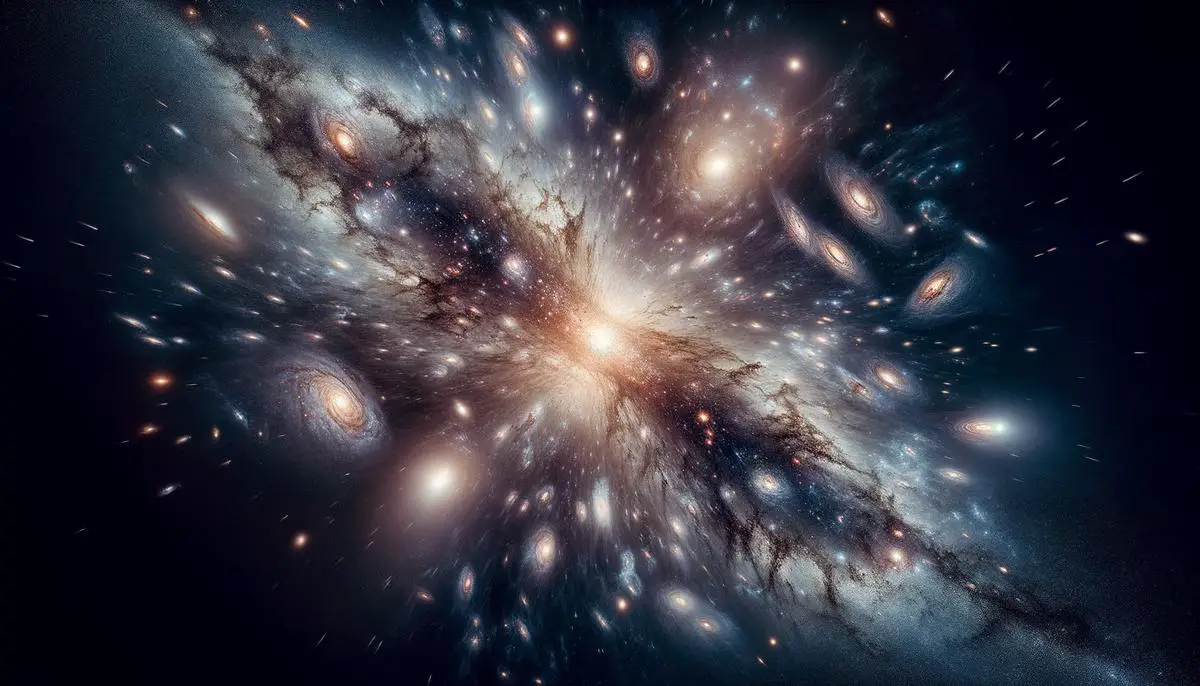
Theoretical Exceptions to Light Speed
Consider for a moment the fanciful realms of theoretical physics, where imaginations about wormholes, warp drives, and other astonishing notions run wild. Could they offer a route around, or rather through, the ironclad speed limit set by light?
Wormholes, a beloved feature in the landscape of popular science fiction, might be visualized as tunnels through the fabric of space and time, connecting two distant points through a shorter route than traditional space travel would permit. Such anomalies are solutions not merely invented for the narrative convenience of travel in galaxies far, far away but arise from real equations under general relativity proposed by Einstein and physicist Nathan Rosen. These 'Einstein-Rosen bridges' underscore a tantalizing yet elusive possibility of linking distinct regions of spacetime, offering a journey that could transcend light speed in practical terms. However, the existence of such portals between cosmos points remains a speculative arena and laden with lurking enigmas neighborly to utter impossibility.
Similarly, warp drives steer the sciencefictional vehicle with theories surmising superluminal — faster-than-light — travel but navigated within accepted boundaries under relativity's guidelines. Coined initially by physicist Miguel Alcubierre in 1994, the warp drive comprises a concept wherein space-time around a spacecraft is theoretically contracted in front and expanded behind. This shifts the craft through space-time, essentially tantalizing with traveling faster than light without violating special relativity. The hurdles faced here pivot upon issues like achieving negatively-energy-based matter and hypervast amounts of regular energy.
This explorative duo of phenomena—wormholes and warp drives—teeter on a sharp edge between tantalizing theoretical prospect and overwhelming practical impedance. Skepticism from the scientific community persists robustly since manipulating space-time on these scales sails directly upstream against an exceedingly strong current of excessive energy prerequisites and cosmic stability requirements.
Venturing into these almost fantastical inquiries does far more than fuel bedtime stories or stir blockbuster cinematic plots; they sharp-focus the demarcation between our current scientific jurisdiction and the hazy territories cloaked just beyond our empiric reach. Tempering our giant theoretical leaps with patient persistence, tenacious research, and a commitment to perennial education promises a gradual yet exciting encapsulation of whatever truths lie patiently waiting beyond these cosmic confines.
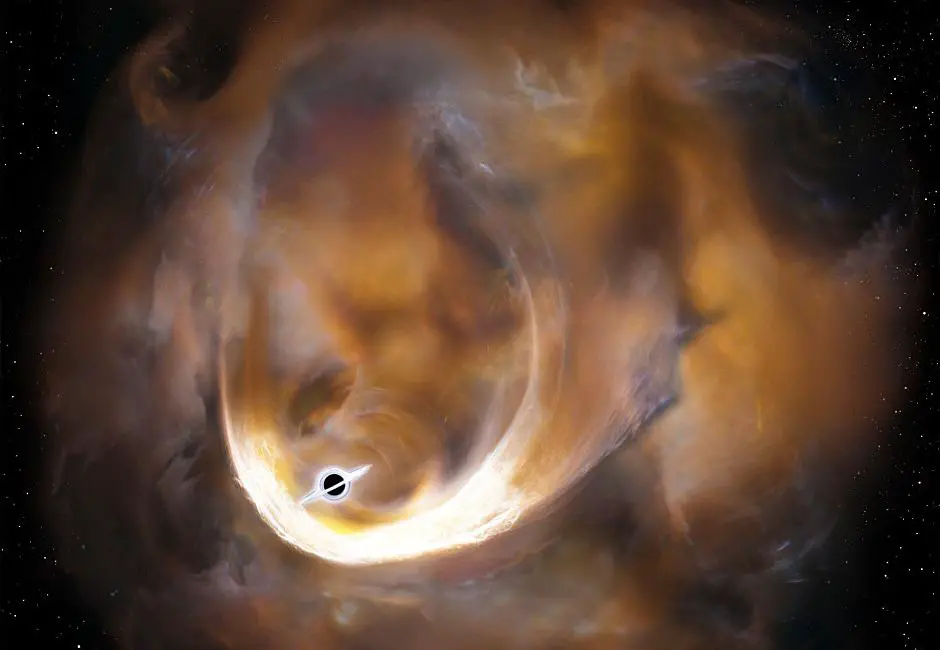
In summary, while the speed of light remains an unbreachable barrier for material objects, it continues to illuminate paths in both theoretical physics and practical technology. The constant velocity at which light travels through the vacuum of space defines the boundaries of speed itself and enhances our understanding of the universe's expansive nature and its underlying frameworks.
- Riess AG, Filippenko AV, Challis P, et al. Observational evidence from supernovae for an accelerating universe and a cosmological constant. Astronomical Journal. 1998;116(3):1009-1038.