Early Solar System and Nebula
4.6 billion years ago, our solar system began as a cloud of gas and dust called the solar nebula. A nearby supernova's shockwaves caused this cloud to collapse and spin, eventually forming our solar system.
Gravity played a crucial role, drawing elements inward. Denser materials gathered at the core, forming the Sun through nuclear fusion. The remaining material created a rotating disk around the Sun, consisting mostly of hydrogen and helium, with some heavier elements scattered throughout.
Smaller particles began sticking together, forming larger clumps called planetesimals. These grew into protoplanets through accretion, gradually pulling in more gas and dust.
Competing Models of Planet Formation
- Core Accretion Model: Suggests planetesimals clumped together over millions of years
- Disk Instability Model: Proposes that clumps of gas and dust collapsed directly to form planets
- Pebble Accretion Theory: Suggests tiny, pebble-sized objects played a crucial role in rapid planet formation
While the Sun formed, other parts of the nebula were creating proto-Earth and other planets. Dense material sank to the core, while lighter material rose to the surface, creating Earth's crust.
Recent evidence suggests Earth's water was present from the beginning, bundled into icy particles during the planet's formation. About 100 million years after Earth's formation, a collision with a Mars-sized body created debris that formed the Moon.1
Volcanic activity released gases that Earth's gravity captured, forming our early atmosphere. The Goldilocks zone, where Earth sits, allowed liquid water to form and remain on the planet's surface.
Asteroids and comets contributed additional water and organic materials to Earth's crust, laying a chemical foundation for life.
Core Accretion and Disk Instability Models
The core accretion model and disk instability model offer two explanations for planetary formation.
Core Accretion Model
This model suggests that nebula particles gradually formed pebbles, boulders, and planetesimals. Over millions of years, these clumped together to form protoplanets. It explains the formation of rocky planets like Earth but struggles to account for the rapid formation of gas giants.
Disk Instability Model
This model proposes that sections of the protoplanetary disk quickly coalesced under their own gravity, forming planet-sized masses almost instantaneously. It better explains the existence of gas giants, which needed to form quickly to capture lighter gases before they dissipated.
Both models face challenges:
- Core accretion struggles with the time required for gas giant formation
- Disk instability may oversimplify complex processes and fails to account for the intricacies of smaller, terrestrial planet formation
The pebble accretion model attempts to bridge these gaps, suggesting that planet formation involved both gradual and rapid processes. It emphasizes the contribution of smaller particles in accelerating planetesimal growth.
As scientists gather more data on exoplanets and their systems, our understanding of planetary formation continues to evolve. These theories not only help us uncover Earth's story but also provide insights into the formation of countless worlds across the universe.2
Rapid Formation and Pebble Accretion
Recent research suggests Earth may have formed more quickly than previously thought, emphasizing the swift accumulation of millimeter-sized pebbles. This theory refines our understanding of planetary genesis, moving away from slower models of the past.
Evidence from chemical signatures in meteorites and ancient planetary bodies supports this rapid formation theory. Analysis of silicon isotopes indicates that Earth incorporated these elements quickly, likely through efficient pebble accumulation.3
Implications of Pebble Accretion Theory
- New perspective on Earth's water origin
- Increased possibility of habitable planets
- Potential universality of rapid planet formation
The pebble accretion theory also offers a new perspective on the origin of Earth's water. The dusty pebbles that formed Earth carried not only silicates but also ice. As these icy pebbles were swept up by proto-Earth's gravity, they brought water with them. This suggests that water was an inherent part of Earth's formation rather than a result of later cosmic collisions.
"If water-carrying pebbles are common in planet formation, many Earth-like worlds could possess the essential ingredients for life."
Observations of distant, young star systems with similar disks of dust and gas hint that rapid planet formation could be a universal phenomenon. Advanced instruments like the James Webb Space Telescope provide critical data on the migration of icy pebbles within planetary disks, supporting the pebble accretion theory.4
As we continue to study Earth's ancient history and observe distant planetary systems, our understanding of planet formation evolves. This research not only alters timelines but also reshapes our perspective on the early solar system's dynamics and the potential for life-supporting planets throughout the universe.
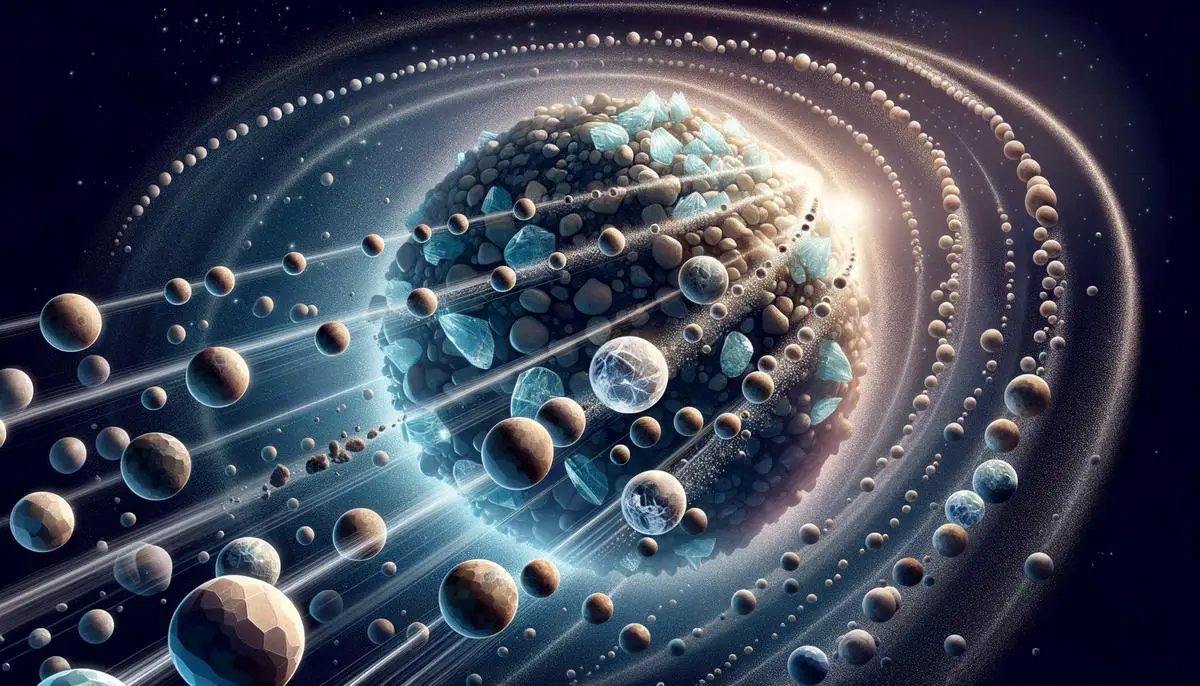
Formation of Earth's Layers
As Earth evolved, its internal structure differentiated into distinct layers through cooling and transformation. This process, known as planetary differentiation, shaped Earth's geological properties.
Initially, proto-Earth was a molten mix of rock and metal, bombarded by asteroids and planetesimals. As it cooled, denser materials sank towards the center, while lighter materials rose to the surface, forming Earth's core, mantle, and crust.
Earth's Layers:
- Core: Divided into solid inner and liquid outer parts, mainly composed of iron and nickel.
- Inner core: About 1,220 kilometers thick, solid due to immense pressure.
- Outer core: 2,260-kilometer-thick layer of molten metal, generates Earth's magnetic field.
- Mantle: Earth's thickest layer at about 2,900 kilometers. Composed mainly of silicate minerals rich in iron and magnesium.
- Crust: Earth's outermost shell, consisting of two types:
- Less dense continental crust
- Denser oceanic crust
The differentiation of Earth's layers set the stage for complex processes that sustain life. By driving plate tectonics, regulating surface temperatures, and creating Earth's protective magnetic field, these layers have continually influenced our planet's habitability.
Understanding Earth's layer formation informs us about our world's history and aids in interpreting the geology of other rocky planets and moons. This knowledge enhances our quest to discover potentially life-supporting planets elsewhere in the cosmos.
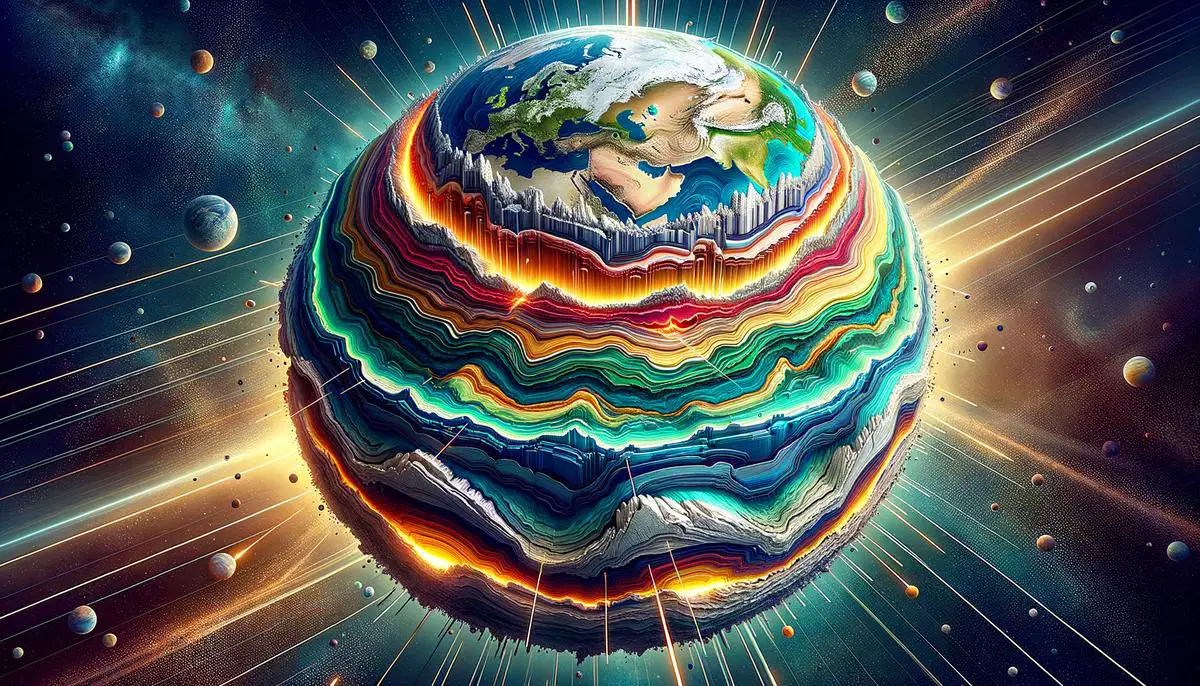
Water and Atmosphere Formation
The acquisition of water and formation of Earth's atmosphere were crucial in transforming our planet into a cradle for life.
Water Acquisition:
- Icy particles captured by Earth's growing gravity
- Impacts from water-rich comets and asteroids
- Volcanic outgassing releasing water vapor
As the surface cooled, water vapor condensed to form oceans. The continuous volcanic activity maintained a steady supply of gases, creating a dynamic balance and influencing atmospheric composition.
Atmosphere Evolution:
- Initial thin atmosphere of hydrogen and helium stripped away by solar wind and heat
- Secondary atmosphere formed through volcanic outgassing and impacts
- Cooling Earth allowed water vapor to accumulate and precipitate as rain
- Emergence of photosynthetic life forms around 3.5 billion years ago
- Great Oxidation Event led to oxygen buildup, enabling complex aerobic life forms
Today, volcanic activity continues to influence our atmosphere, demonstrating the ongoing interplay between Earth's interior and its gaseous envelope. This delicate balance, maintained over billions of years, underscores our planet's resilience and dynamism.
"Understanding the origins of Earth's water and atmosphere provides valuable insights into the conditions necessary for habitability, guiding our search for potentially life-supporting exoplanets."
Implications for Exoplanets
Earth's formation provides a blueprint for understanding the potential for water and habitability on exoplanets. By studying the processes that influenced our planet, we can extend these models to investigate other worlds.
Key Concepts in Exoplanet Research:
- Pebble accretion model: Suggests water might be common on rocky planets
- Disk instability model: Helps understand gas giant formation
- Great Oxidation Event: Provides insight into potential biological activity
- Goldilocks zone: Crucial for identifying potentially habitable planets
- Planetary differentiation: Offers clues about geophysical processes on exoplanets
The pebble accretion model suggests that water might be common on rocky planets. If icy pebbles contributed water during Earth's formation, similar processes could occur elsewhere. Observations from missions like the James Webb Space Telescope support this idea, revealing water vapor in planet-forming disks around young stars1.
The disk instability model helps us understand gas giant formation around other stars. The rapid formation of gas giants could influence the migration and accretion of rocky bodies, potentially moving them into habitable zones where liquid water could persist2.
These planetary formation models offer dual paths to assess exoplanet habitability: the presence of gas giants in a system might indicate dynamic processes that could also facilitate the creation of Earth-like worlds.
As we continue to explore the cosmos with advanced technologies, our knowledge of Earth's blueprint serves as a powerful tool in the quest to discover other potentially habitable planets.
Understanding Earth's formation offers valuable insights into the potential for life on other planets. By examining the processes that shaped our world, we gain clues about the conditions that might make other planets habitable. This knowledge fuels our quest to find other worlds that could support life, extending our understanding of the universe and our place within it.