The journey into the Earth's core is a narrative filled with scientific intrigue and geological marvels. It's a story that connects the very ground beneath our feet to the vast, protective magnetic field that shields our planet.
Core Formation Theories
At the heart of Earth's inner workings is the solid inner core, a Pluto-sized sphere of iron that endures pressures millions of times greater than those experienced on the surface. Scientists have developed theories to explain how this central feature came to be. Initially, Earth was a hot, disorganized mess of material that gradually differentiated into layers through a process known as planetary differentiation. Over time, the heaviest elements sank towards the center, creating the early foundations of the core.
Understanding this process in greater detail relies on the indirect study of seismic waves. As earthquakes occur, they send vibrations rippling through Earth's interior. By studying how these waves speed up, slow down, or change direction, scientists get a peek into the structure of our planet's depths. Findings suggest a core with two distinct parts: a solid inner ball and a swarming, molten outer shell.
Modern research introduces fascinating methods akin to holding a magnifying glass to Earth's earliest years. Laboratory experiments have taken center stage in this exploration. Scientists reproduce the intense conditions of the core by squeezing tiny samples of iron between diamonds and zapping them with lasers. Through these experiments, they've observed how iron's properties change under immense pressures and temperatures, offering clues about the core's age and evolution.
Researchers have also brought the role of the geodynamo to light. This enormous generator powers Earth's magnetic field, a shield deflecting harmful cosmic and solar radiation. The process kicks into gear with the churning movement of the liquid outer core, which then animates the magnetic field enveloping Earth. The crystallization of iron in the solidifying inner core releases latent heat, further fuelling the outer core's convective motions.
Current methods point towards an inner core age of roughly 1 billion to 1.3 billion years. This aligns with geological evidence suggesting a significant strengthening of Earth's magnetism around this time. The thickening inner core could have intensified the geodynamo action, giving our planet's magnetic field a notable boost.
Studying the core also offers scientists analogs for examining other celestial bodies that lack magnetic fields and lead vastly different space lives.
The ongoing evolution of theories about Earth's core underscores not just the core's complexity but also the inventive ways scientists are unraveling its centuries-old secrets. Each experiment and seismic wave analysis adds a piece to the puzzle, drawing us closer to comprehending the full spectacle of Earth's internal dynamics and its protective magnetic embrace.
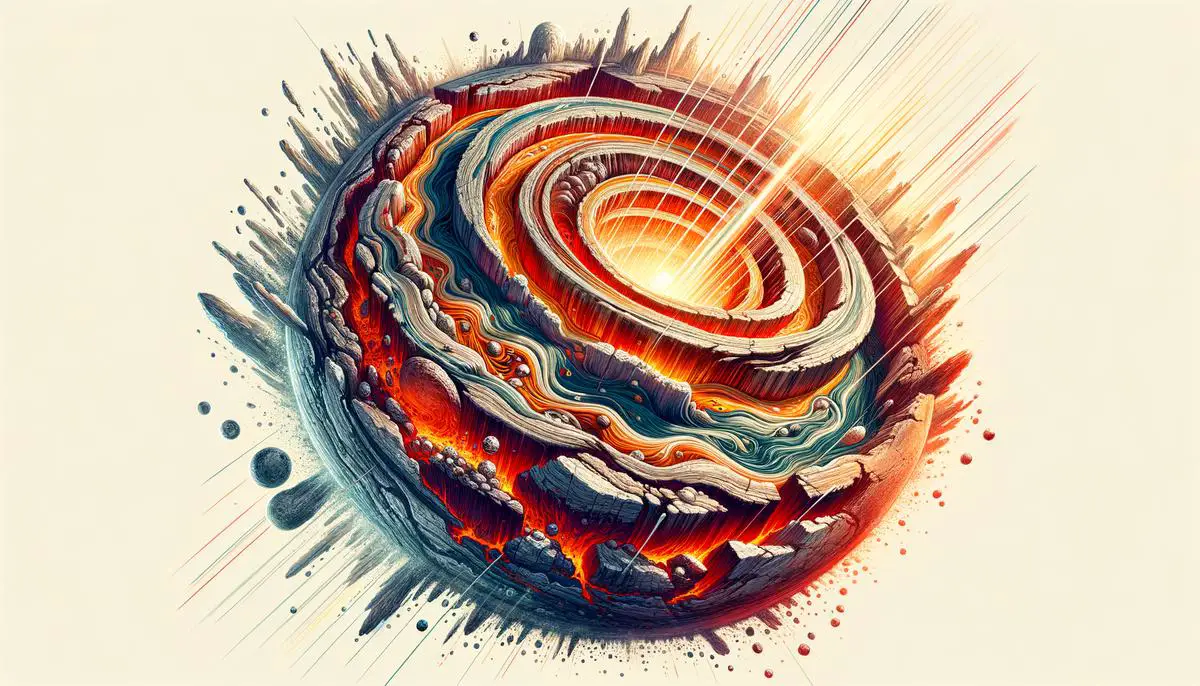
Inner Core Age Debate
The debate over the age of Earth's inner core is punctuated by a spectrum of scientific opinions ranging from a relatively young half a billion years to an ancient lineage stretching over four billion years. This disparity in estimates holds the key to unlocking deeper understandings of Earth's magnetic history and the core's pivotal role within it.
Recent endeavors into this debate have turned towards innovative methods, notably the use of laser-heated diamond anvil cells. This technique allows scientists to simulate the extreme pressures and temperatures akin to those found at Earth's center. By subjecting a miniscule piece of iron—the core's principal constituent—to this infernal environment, researchers can glean insights into the behavior of core materials under such conditions.
This avant-garde approach has shed light on vital parameters, such as the thermal and electrical conductivity of iron at core-like pressures and temperatures. The experimental data drawn from these diamond anvil cell experiments serves as a cornerstone for building models that estimate the age of the inner core based on its cooling history and the energy needed to sustain the geodynamo over time.
One compelling revelation from these studies is the proposition that Earth's core is relatively young in geological terms, aged somewhere between 1 billion to 1.3 billion years. This timeframe coincides with geological markers indicating a significant amplification of Earth's magnetic field strength. The crystallization process of the solid inner core, as it separates from the liquid outer core, releases latent heat, which then energizes the convective movements necessary for the geodynamo. This phase of enhanced geodynamo activity could explain the contemporaneously recorded boost in magnetism, offering a direct link between the inner core's crystallization and the magnetic shield that plays a critical role in maintaining Earth's habitability.
Yet, the inner core age debate is far from settled. Each new method brings its own revelations but also underscores the complexities of replicating and understanding Earth's deep interior processes. The variation in age estimates highlights this challenge and points to the nuanced evolution our planet has undergone. Different components of Earth's core—ranging from its chemical composition to its dynamic conduct—offer a mosaic of evidence that complicates our understanding of geophysical processes.
This scientific journey uncovers more than just the past; it propels us into future explorations about Earth and beyond. By deciphering our planet's magnetic history, researchers pave the way for comparative studies involving other celestial bodies, enriching our comprehension of planetary evolution and the diversity of cosmic phenomena.
As we stand on this frontier, poised between known paths and those veiled in mystery, the quest to pin down the age of Earth's inner core encapsulates the enduring allure of geoscience—its ability to blend ancient riddles with cutting-edge technology, leading us to continuously refine our grasp of the planet's enigmatic heart.
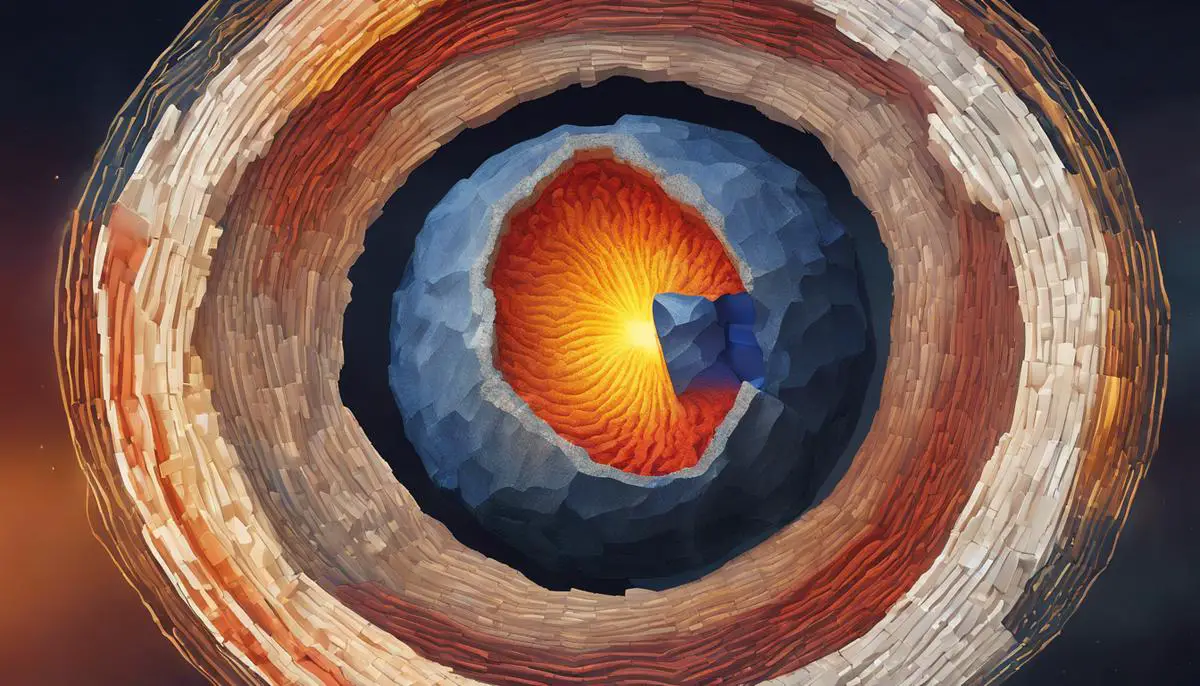
Geodynamo Mechanism
The very essence of Earth's livelihood, its magnetic cloak, is orchestrated by a process hidden deep beneath the surface, an enigma known as the geodynamo. This intricate mechanism involves the relentless movement of liquid iron within the planet's outer core, a ballet that underpins the generation of Earth's magnetic field. The dance between thermal and compositional convection within this liquid iron sea not only fuels the magnetic field but is also subject to the profound influence of the solidifying inner core.
In the depths of Earth's outer core, thermal convection acts as the initial impetus for the geodynamo. As the core cools, less dense, hot liquid iron rises, while denser, cooler iron sinks, initiating a churning movement driven by this heat exchange. This convective motion of electrically conductive fluid generates and sustains Earth's magnetic field. However, the plot thickens with the introduction of compositional convection—a phenomenon tightly intertwined with the crystallization of the inner core.
As iron solidifies at the inner core boundary, it releases lighter elements back into the liquid outer core. This not only contributes additional buoyant force but also infuses the outer core with a compositional gradient, further fueling the convective turmoil. This complex interplay between thermal and compositional forces heightens the efficacy of the geodynamo, ensuring the magnetic field's robustness.
The turning point in this narrative arrives with the solidification of the inner core. This pivotal event supercharges the geodynamo by increasing convection's efficiency. The release of latent heat and lighter elements during crystallization acts as a catalyst, invigorating the geodynamo with a newfound intensity. It's a testament to Earth's dynamism; a silent yet potent transformation echoing through millennia, strengthening the magnetic shield that protects civilizations from cosmic threats.
Earth's magnetic field serves as our planetary guardian, an invisible fortress that extends far beyond the atmosphere. This magnetic shield deflects charged particles from solar winds and cosmic radiation, phenomena that can strip away our atmosphere and erode the conditions necessary for life. Without the relentless churn of liquid iron in the outer core, powered by both heat and composition, Earth's magnetic field would falter, exposing the planet to these celestial assailants.
The enduring strength of this geomagnetic shield also speaks to Earth's uniqueness in our solar system. The magnetic fields of other planets either wane in comparison or are altogether absent, highlighting Earth's fortunate confluence of conditions favorable for life. It underlines how the geodynamo is not just a deep-seated generator of magnetism but a linchpin in the grander scheme of planetary habitability.
As researchers continue to unravel the mysteries of the geodynamo, they are not merely charting the invisible forces that shield our world. They are unwrapping the layers of complexity that define our planet's uniqueness in the cosmos. From thermal and compositional convection to the symbiotic relationship between solidification and convection intensity, each discovery propels our understanding forward, painting a clearer picture of the protective embrace that fosters life on Earth. The act of peering into Earth's metallic heart, thus, extends beyond mere scientific inquiry—it reaffirms our planet's extraordinary aptitude for nurturing life against cosmic odds.
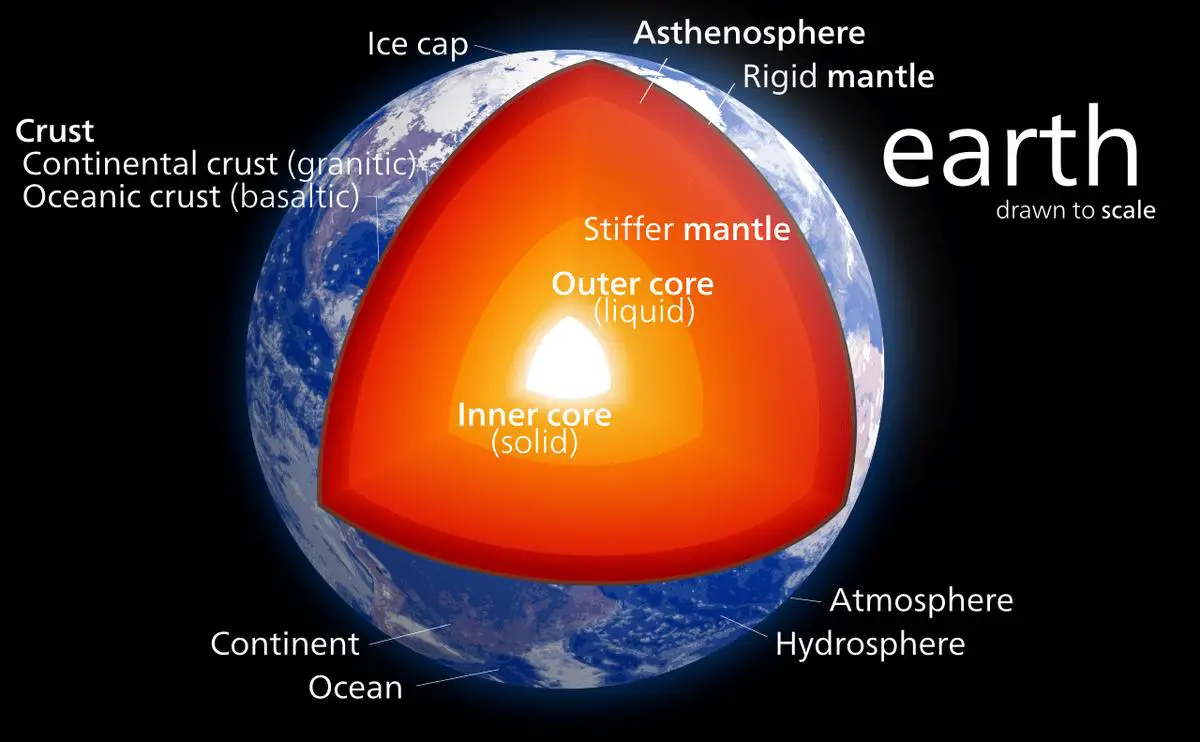
Core's Impact on Surface Dynamics
The intricate processes that unfurl in the depths of Earth's core do not exist in isolation. They reach across the layers of our planet, influencing, and even driving, the dynamic phenomena we observe on the surface. One might wonder how these deep, sequestered events within the inner and outer core can resonate so powerfully across the mantle's expanse, reaching up to touch Earth's crust and atmosphere. The answer lies in the remarkable connectivity of Earth's systems: from the core's intense heat and magnetic field creation to the outer movements of plate tectonics and the fiery eruptions of volcanoes. Each process, though seemingly distant, is interlinked in a global tapestry of geological forces.
The flow of heat from Earth's core to the surface is a prime mover in the ballet of continental drift and the drama of volcanism. Known as mantle convection, this process sees hot material from the deep mantle rise towards the Earth's crust, where it cools, only to plunge back into the depths once more. This mantle conveyor belt is driven by the heat escaping from the core, a continual flow that brews beneath our feet. While the cooling core releases less heat when compared to the mantle's tumultuous currents, it contributes significantly to mantle dynamics, influencing the vigor of plate tectonics and volcanic activity. In regions where this heated mantle material breaches the surface, we witness the spectacle of volcanic eruptions, a powerful testament to the core's hidden influence.
But the conversation between Earth's core and its crust does not end with heat. The magnetic field, generated by the swirling of liquid iron in the outer core, encompasses our planet, reaching far into space to form the magnetosphere. This magnetic embrace has widespread implications not just for compasses and navigation but also for plate tectonics. As plates shift and dive into the mantle at subduction zones, they carry with them records of Earth's magnetic field – a phenomenon known as magnetic striping. These records are crucial for understanding the movements of Earth's plates over geological epochs. Thus, the magnetic whispers from the core echo through time, engraining themselves into the very fabric of the Earth's surface.
Moreover, the magnetic field protects our atmosphere from solar and cosmic wind stripping, a safeguard without which the canvas of life as we know it may have been blank. This protective shield, fostered by the core's restless dynamics, plays its part in regulating Earth's climate over vast timescales. By shielding the atmosphere from solar radiation, the magnetic field indirectly supports a stable environment conducive to a diverse array of life forms and climates.
But could the core's influence extend even further, to sway Earth's climate directly? Some theories suggest that over millions of years, variations in the strength of Earth's geodynamo and thus its magnetic field could influence climate patterns, though this remains a field ripe for further investigation. The discussion weaves together threads of geophysics, climatology, and astrophysics, portraying our planet as a beautifully complex system where core processes resonate with life-sustaining surface dynamics.
In this grand scheme, Earth's core is far more than just a metallic heart; it's a dynamic engine that drives fundamental processes affecting Earth's surface and climate. From forging mountains and carving basins to fostering climates that have allowed life to thrive and diversify, the core's silent, unseen rhythm orchestrates a planetary dance of unparalleled complexity. As we uncover more about these deep connections, we gain not only insights into our world's past but also glimpses of its future, all tied to the pulsing heart of a planet that is alive and dynamic from its core to the sky.
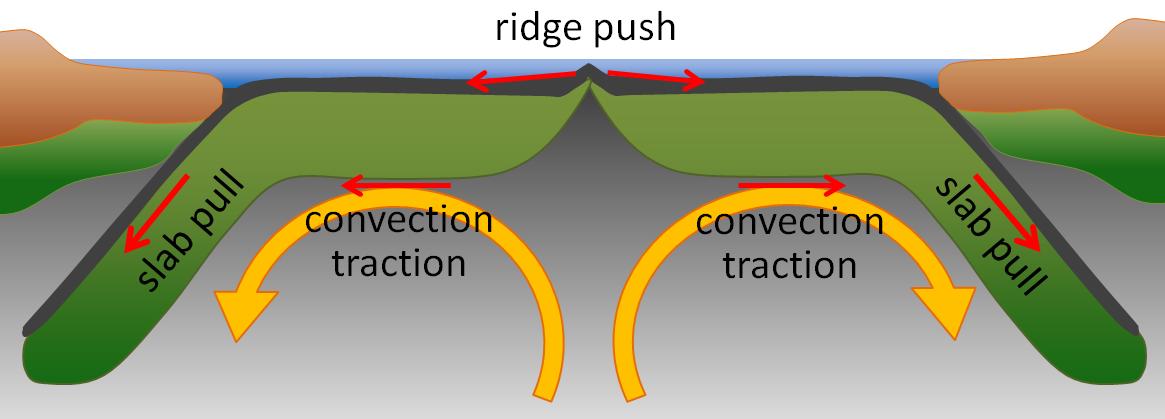
Comparative Planetary Cores
Mercury's oversized metallic core, spanning about 85% of its planetary radius, differs greatly from Earth's. The immense size of Mercury's core suggests a different cooling and geological history, possibly offering clues about the planet's dense formation but leaving it without the protective magnetic field necessary to shield a potential atmosphere from solar winds.
Venus, similar to Earth in size and mass, has a core that might be partly liquid. However, Venus lacks an intrinsic magnetic field, which significantly impacts its ability to retain an atmosphere conducive to life, highlighting Earth's unique protective magnetic field.
Mars once had a churning core that produced a dynamo effect similar to Earth's, but it has since ceased, allowing its magnetic field to dissipate. Evidence of ancient stream beds and mineral deposits that form in the presence of water suggest Mars once had more habitable conditions. The cessation of its planetary dynamo has led to a thin atmosphere, exposing the surface to harmful solar and cosmic radiation.
Jupiter and Saturn have cores potentially composed of rock, metal, and hydrogen compounds under extremely high pressures. These cores contribute to the generation of their strong magnetic fields, which are vastly different from Earth's and serve purposes like herding auroras but do little to make their environments more hospitable.
Neptune and Uranus have icy cores surrounded by slushy mantles of ammonia, methane, and water. Their magnetic fields, significantly tilted from their rotational axes, hint at complex inner dynamics different from the traditional core dynamo theory exemplified by Earth. While these mysterious interiors are fascinating, they emphasize the uniqueness of Earth's inner structure in supporting life.
Exoplanets orbiting other stars present the possibility of core compositions we haven't yet discovered. However, our understanding of Earth's core provides a foundation for speculating about exoplanetary habitability. The formation of a magnetic field capable of redirecting stellar wind and maintaining a stable atmosphere seems crucial, similar to Earth's advantageous configuration.
Earth's core arrangement fosters a magnetic field that protects our planet and makes it a haven for life. This intricate relationship between Earth's core and its surface is a critical factor that makes our planet habitable amidst the vastness of space. As we continue to explore the cores of other worlds, we gain a deeper appreciation for the delicate balance that sustains life on Earth, highlighting our planet's core as a vital component for habitability.
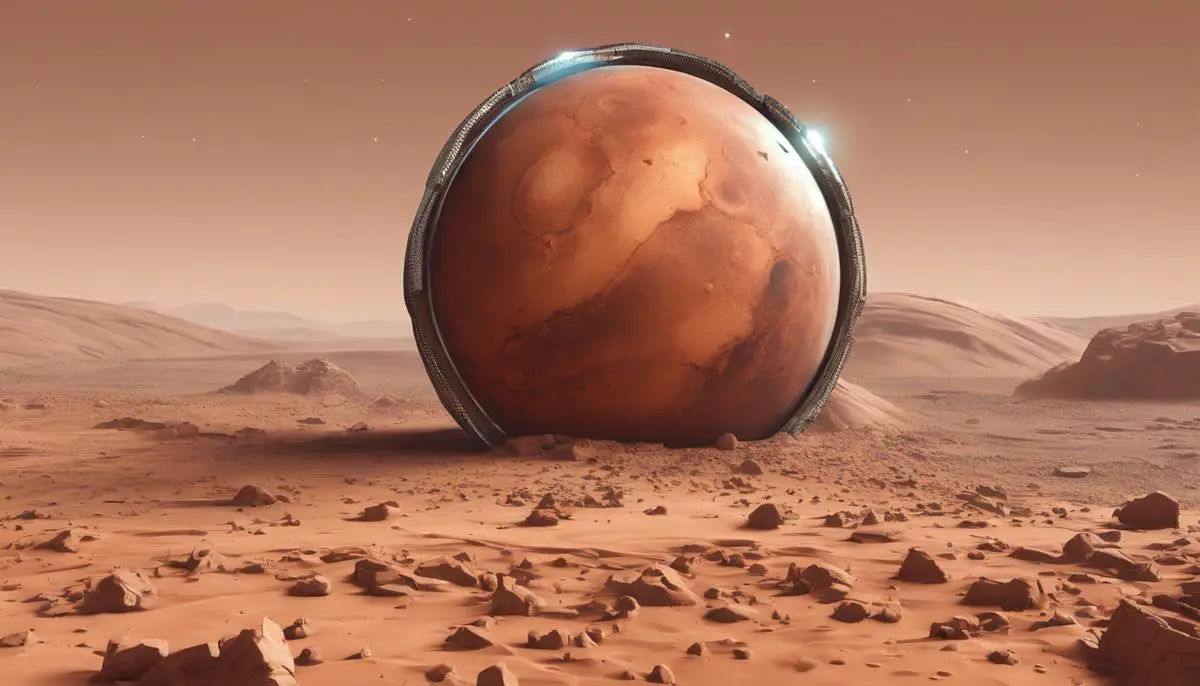
The core of our planet is more than just a metallic sphere; it is a crucial component in a series of dynamic processes that protect life on Earth. The generation of the magnetic field, resulting from the geodynamo effect within the liquid outer core, demonstrates Earth's remarkable ability to sustain life. This protective magnetic field ensures our planet remains a cradle for biodiversity amidst the cosmic expanse, emphasizing the significance of Earth's inner workings.
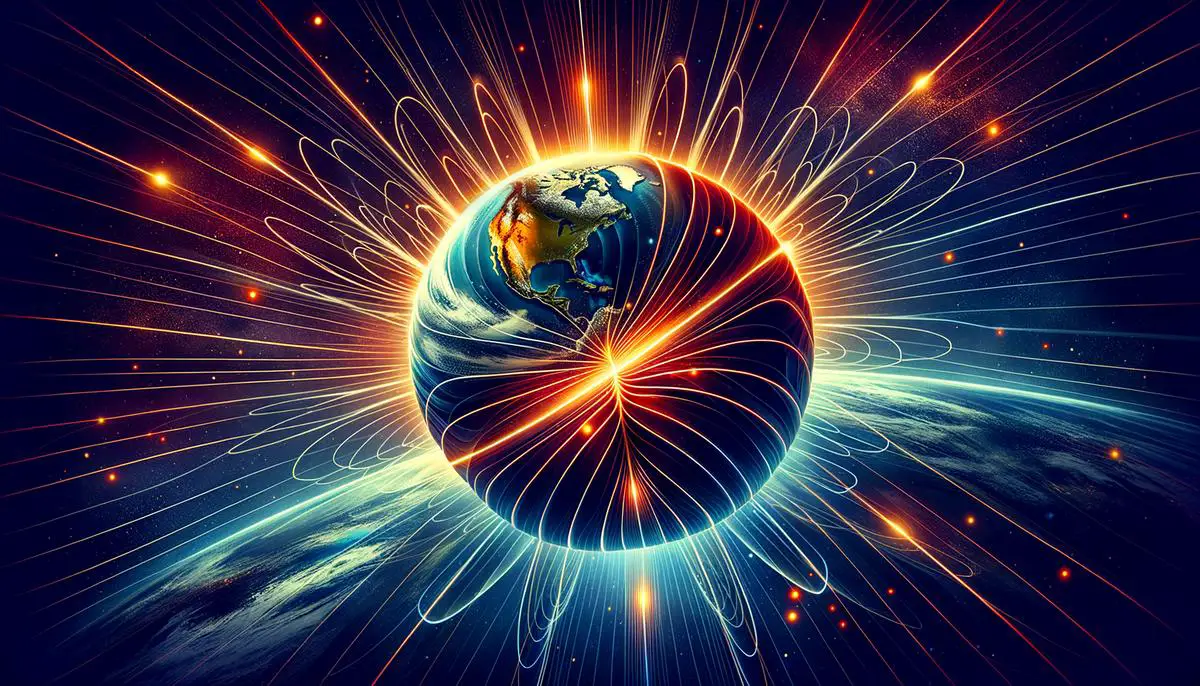