The Nature of Dark Matter
Dark matter, an invisible component of our universe, exerts a profound gravitational influence despite its imperceptibility. It doesn't interact with light or energy, making it undetectable by conventional astronomical tools. However, its gravitational effects are undeniable.
Scientists have proposed several explanations for dark matter's nature:
- Weakly Interacting Massive Particles (WIMPs): Theorized to be much heavier than protons but elusive in their interactions with regular matter.
- Axions: Particles with even less mass than electrons, which could potentially convert into photons under specific conditions.
Dark matter's unique properties extend to its lack of interaction through electromagnetic forces. It neither absorbs, reflects, nor emits light, yet its gravitational influence is crucial in holding galaxies together. Without dark matter, galaxies would likely disintegrate due to their rotational forces.
The search for dark matter's secrets is more than an academic exercise. It plays a vital role in our understanding of galaxy formation, evolution, and the structure of the universe. Scientists employ advanced detectors and space telescopes in their quest to observe dark matter's effects and unravel its mysteries.
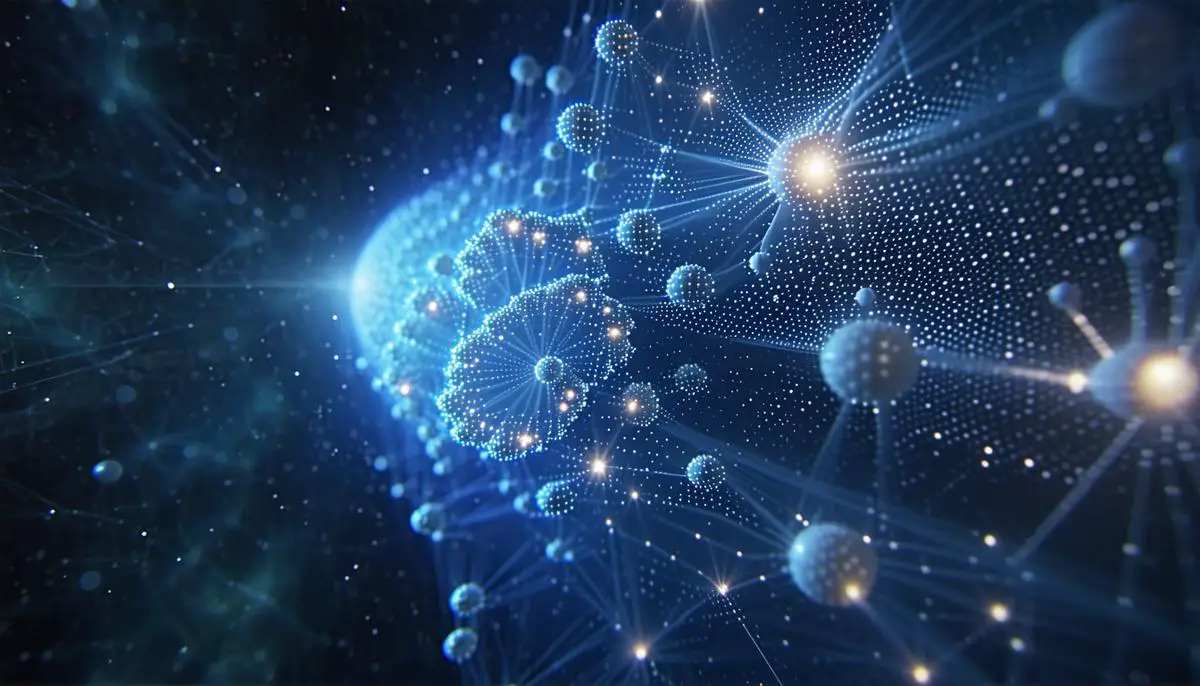
Evidence for Dark Matter
The existence of dark matter is supported by several lines of evidence:
- Galaxy rotation curves: In the 1970s, astronomers discovered that stars at the outer edges of galaxies orbit at speeds inconsistent with the visible mass distribution. This phenomenon suggests the presence of additional, unseen mass – dark matter – providing the necessary gravitational force to maintain these rapid orbital velocities.
- Gravitational lensing: Massive objects, such as galaxy clusters, bend light from distant sources, creating distorted or multiple images. The degree of bending indicates a greater mass than what is visibly present, pointing to the existence of dark matter.
- Colliding galaxy clusters: The behavior of colliding galaxy clusters, such as the famous "Bullet Cluster," provides further support for dark matter. In these cosmic collisions, the bulk of the mass appears separated from the visible matter, a phenomenon best explained by the presence of dark matter.
- Cosmic microwave background (CMB): Analysis of the CMB's tiny fluctuations allows scientists to map the distribution of matter in the early universe, revealing more mass than can be accounted for by visible matter alone.
These varied lines of evidence collectively build a strong case for the existence of dark matter, driving ongoing research to uncover its nature and properties.
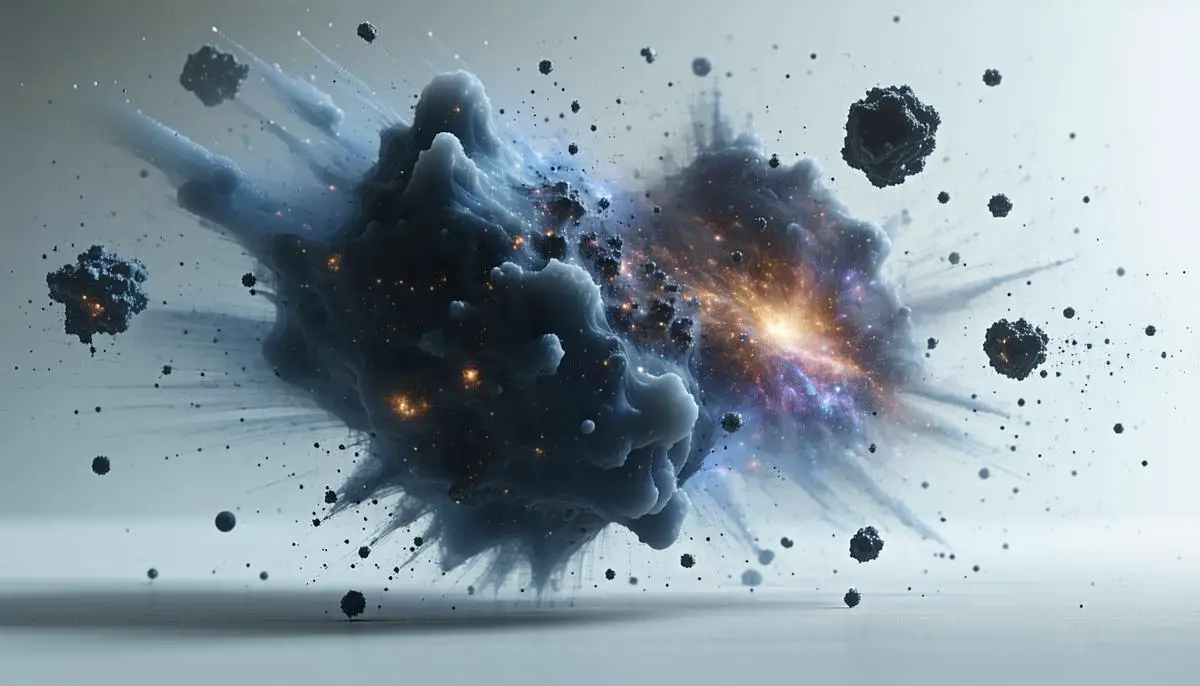
Dark Matter Detection Methods
Scientists employ a variety of sophisticated techniques in their quest to detect dark matter:
- Deep underground detectors: Shielded from cosmic radiation, these aim to capture rare interactions between dark matter particles and ordinary matter. The XENON1T experiment in Italy, for example, searches for collisions between hypothesized WIMPs and xenon atoms.
- Particle accelerators: Facilities like the Large Hadron Collider (LHC) collide particles at extremely high energies, hoping to produce dark matter particles or their effects, even if they can't be directly observed.
- Space-based observatories: The Alpha Magnetic Spectrometer (AMS) on the International Space Station looks for antimatter in cosmic rays, which could indicate dark matter interactions. The European Space Agency's Euclid mission aims to map the universe's geometry, potentially revealing dark matter's gravitational effects.
- Innovative data analysis: Deep-learning algorithms are being developed to recognize dark matter's subtle influences in astrophysical observations. These computational methods may provide new insights into dark matter's behavior and distribution.
Despite the challenges posed by dark matter's elusive nature, the scientific community continues to refine and expand detection methods, driven by the potential for groundbreaking discoveries in our understanding of the universe.
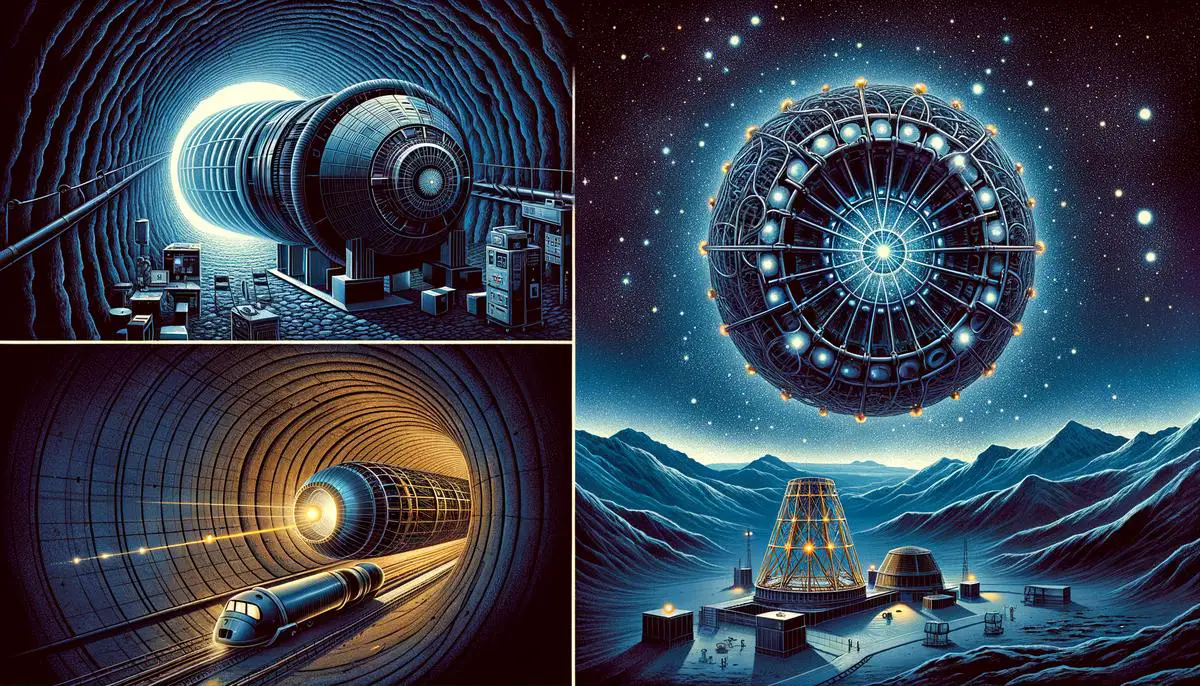
Dark Matter's Role in the Universe
Dark matter plays a crucial role in shaping the structure and evolution of the universe. It acts as a cosmic scaffolding, providing the gravitational framework around which galaxies and larger cosmic structures form and develop.
In the early universe, dark matter was instrumental in seeding the initial density fluctuations that led to the formation of the first stars and galaxies. Its gravitational influence attracted ordinary matter, creating the foundational structures of the cosmic web we observe today.
Dark matter halos surrounding galaxies significantly influence their shape, rotation, and evolution. These halos also facilitate the clustering of galaxies into larger structures, from simple galaxy pairs to vast superclusters.
"Motions of the stars tell you how much matter there is. They don't care what form the matter is, they just tell you that it's there." – Pieter van Dokkum, Yale University
The interplay between dark matter and ordinary matter is evident in phenomena like gravitational lensing, where the bending of light reveals the presence of unseen mass. This effect allows astronomers to map dark matter distributions and study its impact on cosmic structures.
As our understanding of dark matter grows, it continues to reshape our view of the universe's past, present, and future evolution. The ongoing study of dark matter promises to reveal new insights into the fundamental nature of the cosmos and its most enigmatic components.
Theories and Models of Dark Matter
Several theories attempt to explain the nature of dark matter, each with its own strengths and challenges:
- Supersymmetry: Proposes that each known particle has a heavier partner, potentially providing candidates for dark matter particles such as the neutralino. This elegant theory offers a potential solution to multiple cosmological and particle physics questions.
- Extra-dimensional theories: Suggest that dark matter might exist primarily in hidden dimensions, interacting with our observable universe primarily through gravity. These models provide an intriguing explanation for dark matter's elusive nature but present significant challenges for experimental verification.
- Hidden Valley theory: Posits a separate sector of particles interacting weakly with known matter. This concept envisions dark matter as part of a parallel particle world, connected to ours through subtle interactions.
Each of these theories faces substantial hurdles in experimental validation. Supersymmetry requires energies beyond current technological capabilities, while extra-dimensional and Hidden Valley models stretch the limits of observational feasibility.
Despite these challenges, these theoretical frameworks drive innovation in physics and cosmology. They encourage scientists to explore new experimental techniques and observational strategies, potentially leading to breakthroughs in our understanding of dark matter and the fundamental nature of the universe.
As research progresses, these theories may evolve or give way to new models. The ongoing pursuit of dark matter's true nature continues to push the boundaries of physics and our comprehension of the cosmos.
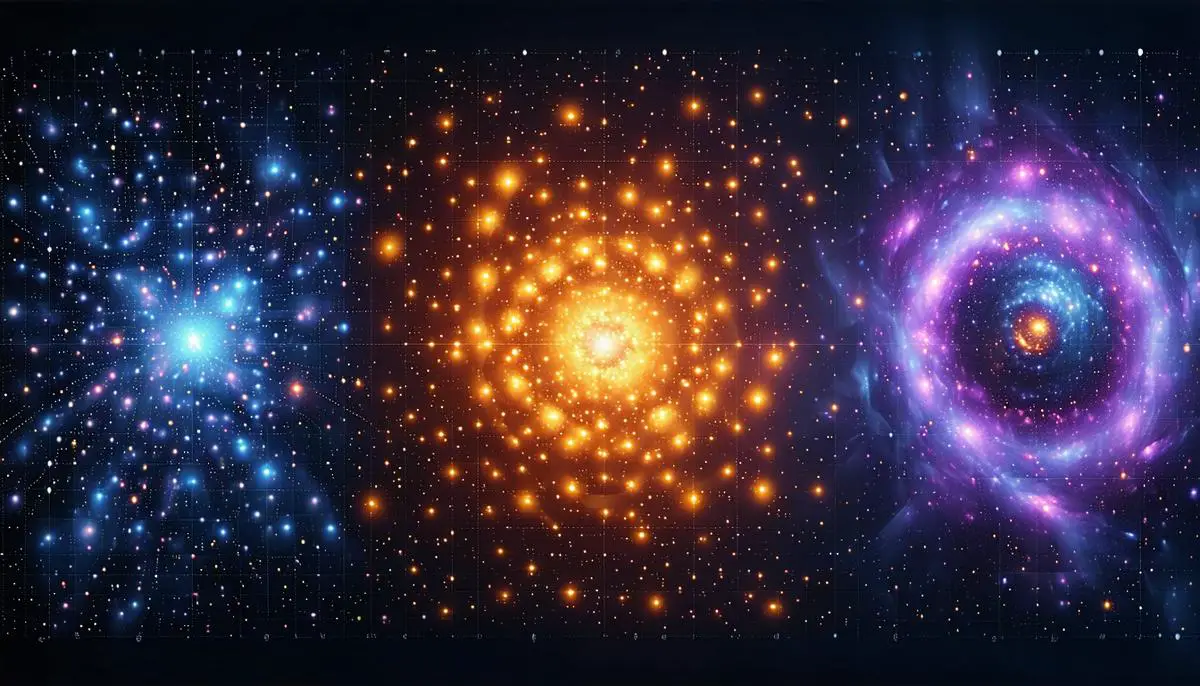
Dark matter, an unseen force shaping the universe, remains a captivating mystery. Its elusive nature challenges our understanding, urging us to unravel its secrets. As we persist in this cosmic quest, the potential for discovery fuels our curiosity and determination. The pursuit of dark matter's truth promises to illuminate the hidden structures of our universe, inspiring us to continue exploring the unknown.
- Zwicky F. Die Rotverschiebung von extragalaktischen Nebeln. Helv Phys Acta. 1933;6:110-127.
- Rubin V, Ford WK Jr. Rotation of the Andromeda Nebula from a Spectroscopic Survey of Emission Regions. Astrophys J. 1970;159:379.
- van Dokkum P, Danieli S, Cohen Y, et al. A galaxy lacking dark matter. Nature. 2018;555:629-632.
- Ting S. The Alpha Magnetic Spectrometer on the International Space Station. Nucl Phys B Proc Suppl. 2013;243-244:12-24.
- Harvey D, Robertson A, Massey R, McCarthy IG. Observable tests of self-interacting dark matter in galaxy clusters: BCG wobbles in a new dark matter regime. Mon Not R Astron Soc. 2019;488:1572-1579.