Lunar dust, a seemingly trivial aspect of the moon’s environment, presents a significant challenge for astronauts and machinery alike. This article sheds light on the various hurdles posed by the lunar landscape, from the abrasive nature of lunar dust to the difficulties of landing and operating on the moon’s surface. By examining these challenges, we gain insight into the innovative solutions and strategies developed to overcome them, highlighting the importance of adaptability and ingenuity in space missions.
Lunar Dust and Its Impacts
Lunar dust, or regolith, behaves differently than dust on Earth. Billions of years without water or wind have left it sharp and jagged, turning tiny grains into abrasive particles that cling to everything. During the Apollo missions, astronauts found their suits eroded by lunar dust after just a few days of exposure. The dust damaged seals, obscured visors, and wore through protective layers of their gear.
This dust isn't just problematic for space suits; hardware and vehicles on the moon face similar challenges. The Chinese Yutu rover had its operations jeopardized by lunar regolith, hinting at a frustrating future for mechanical explorers that could get immobilized by the abrasive substance.
Lunar dust also has the ability to become supercharged and disrupt machinery and health. The moon's lack of atmosphere allows solar UV light to charge the dust, making it adhere to anything with a charge, similar to rubbing a balloon on your hair and sticking it to a wall. This charged dust can fry sensitive electronics and interfere with equipment.
Health risks arise when considering long-term exposure. Apollo astronauts reported "lunar hay fever" from inhaling the tiny particles, which they found inside their landers despite their best cleaning efforts.1 Sharp and minuscule, this regolith can lodge in lungs if inhaled, posing severe risks to astronaut health, akin to concerns around asbestos on Earth.
Combating lunar dust necessitates innovative materials. As no single fabric can tackle regolith alone, teams are developing creative layering solutions to resist dust while maintaining flexibility for astronauts. Projects today focus on testing regimes that subject suit materials to extreme conditions—accelerated aging, UV radiation exposure, and artificial lunar dust tumbles—to find the best defense against lunar wear and tear.
With ambitions to return to and possibly inhabit the Moon, understanding and overcoming the challenge posed by lunar dust is paramount. Establishing a Moon base would involve constant dust kickup, potentially eroding habitats, jamming machines, and endangering lives. Future lunar exploration may require not only gravity boots but also shields against the dusty, abrasive environment that seeks to wear down both spirits and space suits.
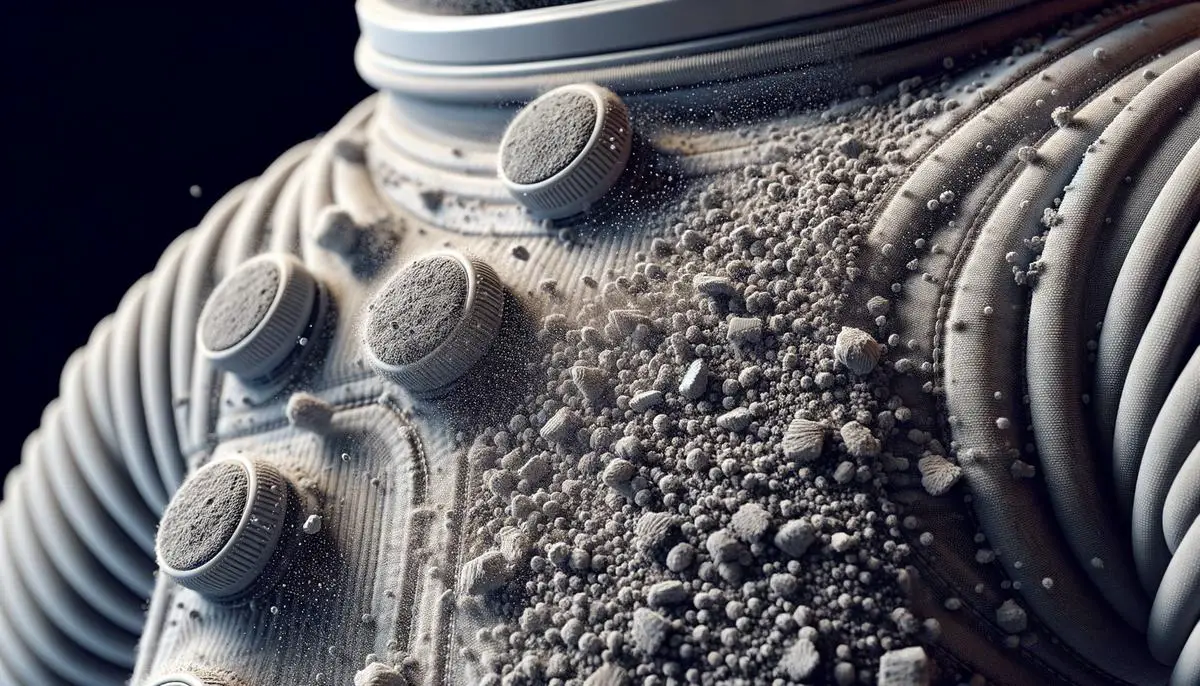
Landing and Navigation Difficulties
The moon's weak gravitational pull, only one-sixth that of Earth's, poses a unique set of challenges for scientists and engineers exploring the lunar surface. This reduced gravity makes landing spacecraft a precarious endeavor, akin to landing a paper airplane on a moving target rather than a jumbo jet on a runway, demanding precision engineering and a thorough understanding of celestial mechanics.
The absence of a substantial atmosphere on the moon means that spacecraft must rely solely on their engines to decelerate and land safely, without the luxury of gliding to a stop as they would on Earth. Maneuvering to a secure landing spot is further complicated by the moon's inhospitable surface, riddled with craters, boulders, and uneven terrain. Each lunar landing mission must carefully select a location that is both scientifically valuable and safe for landing, navigating with meticulous calculations.
The razor-thin margins for error are evident in the failures and near misses of past missions, with the complexities heightened by real-time communication delays between Earth-based mission control and the spacecraft. These delays, though seemingly trivial in everyday life, can be the difference between success and failure when guiding a spacecraft to land on another celestial body.
Lunar daylight, lasting up to 14 Earth days, brings temperatures that can fry electronics and critical spacecraft components, while the extreme cold during the lunar night can freeze and impair technology.2 Equipment brought from Earth must withstand these temperature extremes, which are not encountered on our home planet.
Landing and surviving on the moon demands confronting these daunting realities and outsmarting them. It is not merely a question of whether we can achieve successful lunar exploration, but how we adapt our technologies and strategies to meet and overcome these challenges head-on. Each layer of complexity drives engineers and scientists to innovate, spurring advancements that could one day make lunar and even Martian habitats a reality.
Navigating and exploring the lunar surface is a delicate ballet performed on a stage fraught with unseen perils and unforgiving physics. The moon commands respect and forethought; its seemingly barren landscape presents puzzles that have pushed human ingenuity to its limits, compelling us to innovate, adapt, and overcome.
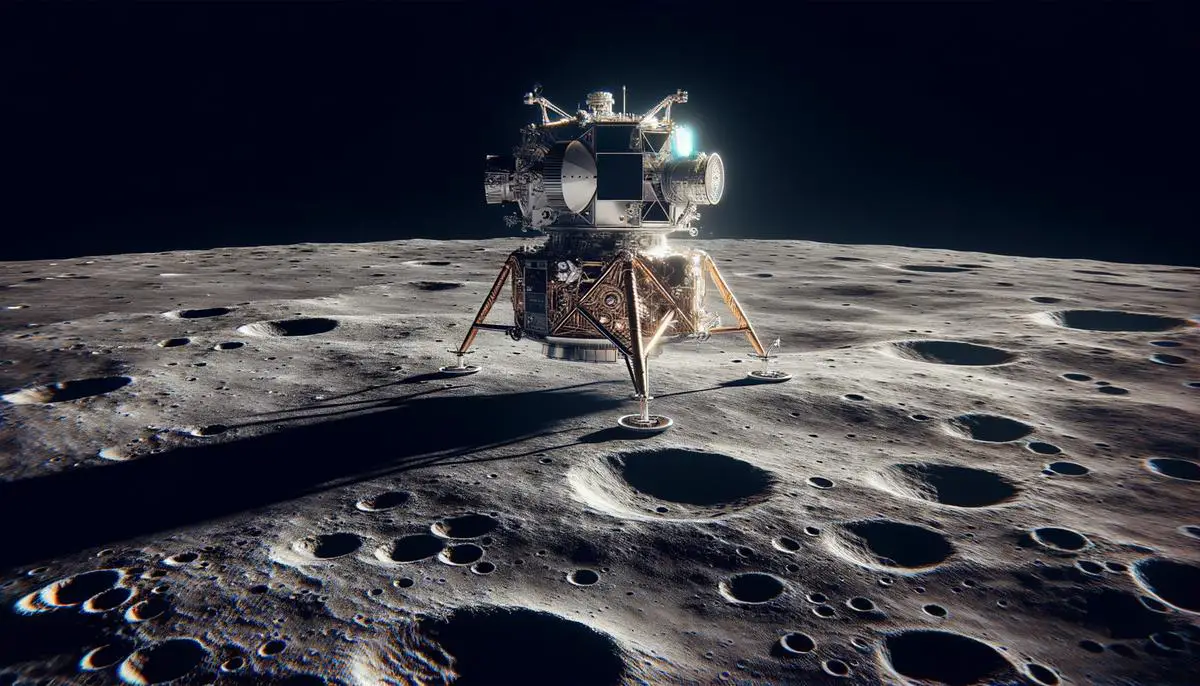
Sustainability of Lunar Missions
Sustainable lunar missions hinge on several key strategies, building upon the groundwork laid by the struggles and solutions related to the moon's conditions. Developing and implementing reusable launch vehicles and landers is a cornerstone of this approach. By designing spacecraft that can return to Earth, undergo refurbishment, and be launched again, space agencies significantly reduce the cost and resources required for each mission. This approach mirrors the recycling ethos found on Earth, extending the life cycle of space hardware and making missions more budget-friendly.
The Artemis program recognizes the indispensability of local resource utilization (LRU), marking a shift from Earth-dependent missions to self-sustaining exploration. Harnessing the moon's resources, such as extracting water ice from permanently shadowed craters to produce drinking water, oxygen, and rocket fuel, transforms the moon from a barren waypoint into a bustling pit stop for spacecraft.
Energy management is another linchpin for sustained lunar visits. Solar power, abundantly available on the moon's surface despite extreme variations in the lunar day and night cycle, offers a clean, reliable energy source. Future missions may deploy vast solar arrays to capture the sun's rays, storing that energy for use during the two-week-long lunar night or in shadier regions.
Advancing robotics and automation on the moon's surface is crucial. Robotic precursors could prepare landing sites, assemble habitats, and conduct science operations autonomously or with remote guidance from Earth, reducing risks for human explorers and increasing setup efficiency before crewed missions land.3 Robots might also maintain bases during the long stretches between human visits, ensuring astronauts step into a functional, welcoming environment rather than a mothballed outpost.
International cooperation is vital, as pooling knowledge, resources, and ambitions leads to more robust missions. The Artemis Accords pave the way for peaceful, collaborative exploration, binding signatory nations to principles that ensure transparency, cooperation, and mutual respect.4 This global perspective fosters shared technological advancements and infrastructure development in orbit and on the lunar surface, akin to Earth's Antarctic research stations, with countries working together in harsh conditions toward common scientific goals.
The renewable and adaptable nature of lunar exploration extends to housing, with plans for habitats that can shelter astronauts from extreme temperatures and radiation. Innovations could include 3D-printed structures using lunar regolith as building material, reducing the need to transport construction materials from Earth and providing practical experience in using off-world resources for construction—a vital skill for deeper space exploration.
Addressing these financial, technological, and logistical challenges with ingenuity and collaboration paves the way for a future where monthly mission launches to the moon might become as routine as international flights are today, each one bringing us closer to untangling the moon's mysteries while laying the foundation for ventures beyond.
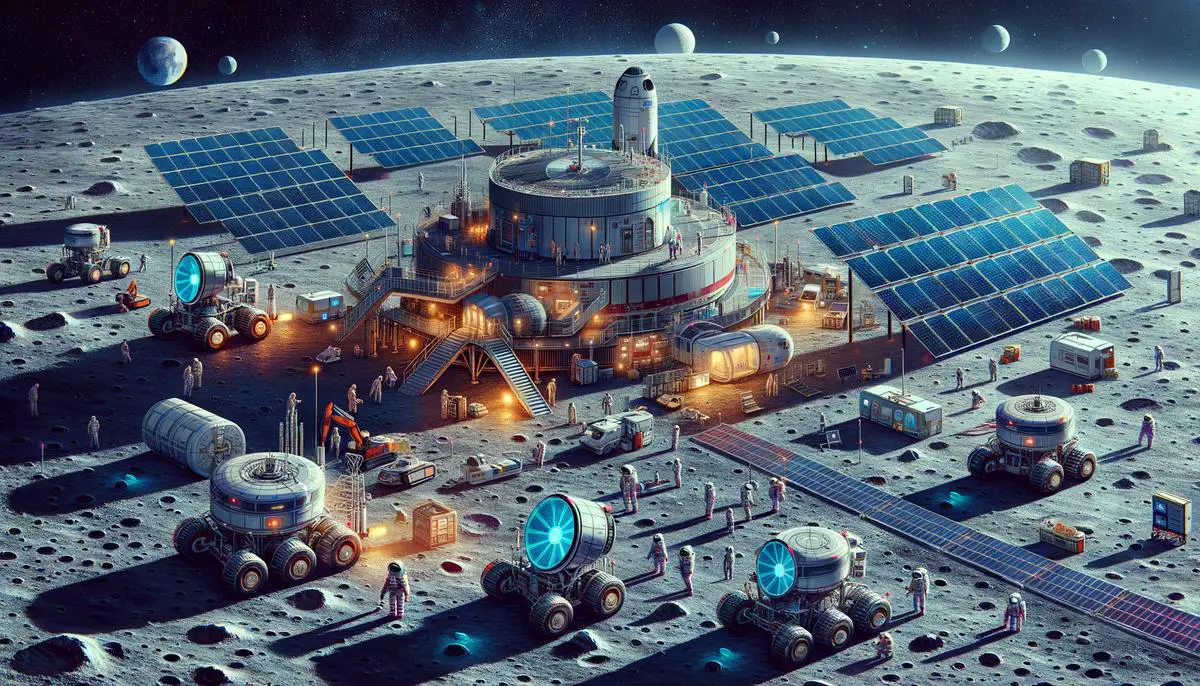
Space Suit Development for Lunar Exploration
Space suits for lunar exploration must offer unprecedented levels of protection and flexibility to handle an environment unlike any on Earth. The moon's extreme temperature fluctuations, ranging from -173°C during the lunar night to 127°C in the daytime, pose a significant hurdle.5 Suit materials need to insulate astronauts from these extremes while allowing for the dissipation of body heat during physical exertion.
Creating a suit that allows for ample mobility is a pressing design challenge. The moon's lower gravity means astronauts can jump higher and farther but also risk losing balance and falling over. Falling in a bulky, rigid suit could endanger the astronaut and damage the suit itself. Suits must be flexible enough to facilitate ease of movement for various tasks, including walking, bending, and picking up objects, while maintaining integrity against the moon's harsh conditions.
Adjusting to the moon's low gravity requires suits equipped with a lightweight and efficient life support system that manages oxygen supply, removes carbon dioxide, and regulates temperature and humidity—all critical for keeping astronauts alive and comfortable.
Visibility is another key factor. The sun's unfiltered rays on the moon's surface can be blinding, necessitating a visor design that protects against intense light and harmful UV radiation. This visor must also combat the absence of atmosphere, which results in darker shadows, making it harder to discern details in shaded areas.
Developing suits that protect against cosmic radiation presents another layer of complexity. The absence of a protective atmosphere on the moon leaves astronauts vulnerable to higher levels of space radiation, which can have serious long-term health implications. This calls for innovative materials that shield astronauts from radiation without significantly increasing the suit's weight or reducing mobility.
Enhancing suit durability is crucial for reducing maintenance and ensuring longevity. Lunar expeditions may involve extended stays, requiring suits that can withstand repeated use over long periods. New sealing technologies must be developed to protect vulnerable spaces in the suit from dust infiltration, as traditional fastening systems like zippers or velcro prove less effective in lunar regolith.
Communication systems integrated into the suits need to be reliable over the lunar expanse. Given the moon's rough terrain and potential for explorers to venture beyond direct line of sight with their lander or base station, ensuring clear and constant communication is critical.
Developing gloves that allow for fine motor control while retaining protection against the lunar elements is challenging. Lunar exploration involves both broad movements like walking or climbing and precise tasks like sample collection or equipment handling, so gloves must be dexterous without sacrificing safety.
Overcoming these challenges involves a blend of materials science, biomechanical engineering, and a deep understanding of human physiology in space. Innovations in fabrics, joint designs, and life-support systems are progressively tackling these hurdles, reflecting human ingenuity in our quest to explore beyond our earthly confines.
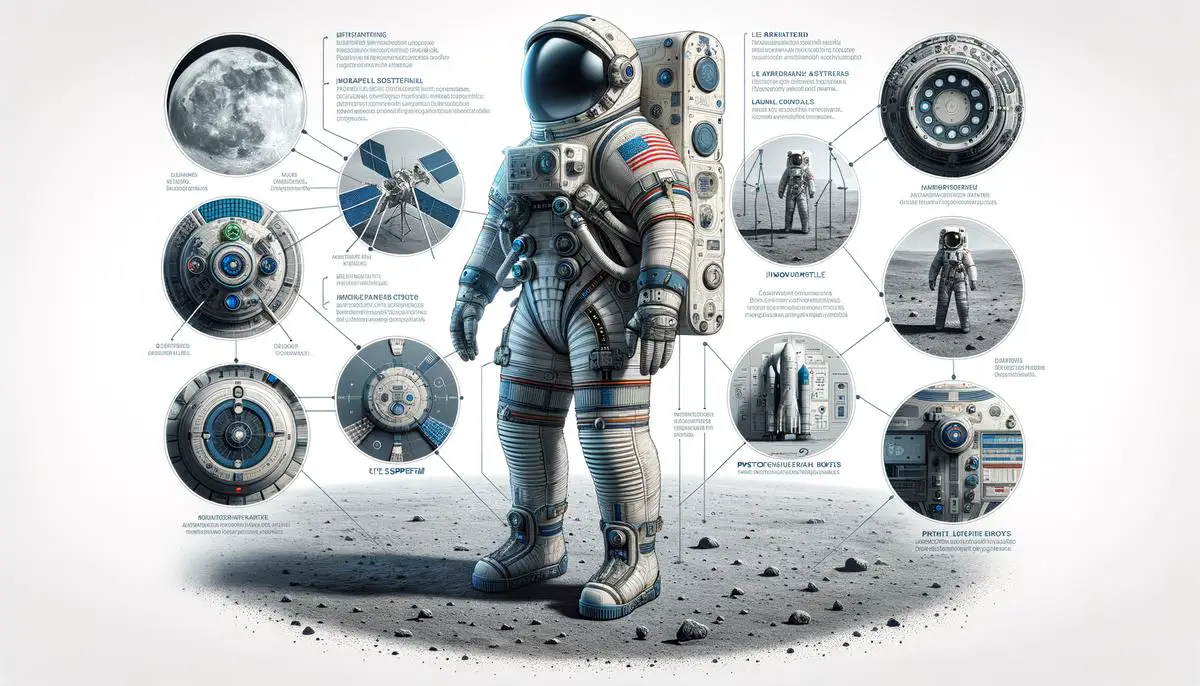
Impact of Lunar Environment on Technology
On the moon, machinery and technology face a harsh reality: radiation from the sun hits the surface relentlessly, posing a severe risk. This constant bombardment can degrade materials and interfere with the delicate internal circuitry of machines, proving that the moon's surface is no friend to unprotected tech.
To combat this, scientists meticulously create shields using materials that can block or absorb harmful radiation. This acts as a suit of armor for sensitive electronics, ensuring that missions don't hit a snag due to fried circuit boards.
The drastic temperature swings – plunging into cold darkness then surging into blazing sunlight – test the limits of both machinery and materials used in lunar missions. Engineers have to smartly choose and sometimes invent new materials that can withstand being roasted and frozen repeatedly without cracking, warping, or losing function. Thermal blankets and specially formulated paints are among the innovations providing these crucial layers of protection.
Maintenance strategies also get creative on the moon. Traditional lubricants turn to dust in the vacuum of space or freeze in cold shadows, so dry lubricants come into play. These keep machinery moving smoothly without attracting and holding onto the ever-persistent lunar dust.
For activities not directly battered by the lunar environment, like some secluded operation bases or shadowed crater explorations, maintaining technology becomes an act of balance. Careful heating elements keep machinery at operational temperatures without letting them get too warm, and sun shields can reflect away just enough light to prevent overheating while still allowing solar panels to drink in the energy they require.
The unrelenting sunlight can also play tricks on visibility. Glare and brightness don't just affect astronaut visors; cameras and sensors need their own "sunglasses." Coatings and filters that reduce glare yet allow for clear data collection are crucial—making sure that missions can see what they're doing and discover what they're there to find.
Cosmic radiation not only impacts human explorers but can errode unprotected tech over time. Innovative radiation-hardened components also secure a frontier for maintaining functionality amidst cosmic ray showers.6
Navigating these extremes demands technologies that are exceptionally robust yet surprisingly nimble. These strategies and innovations embody humanity's unyielding drive to explore, unveiling the harsh beauty of the lunar landscape while ensuring that the delicate sinews of our ambition remain intact and operational throughout the journey.
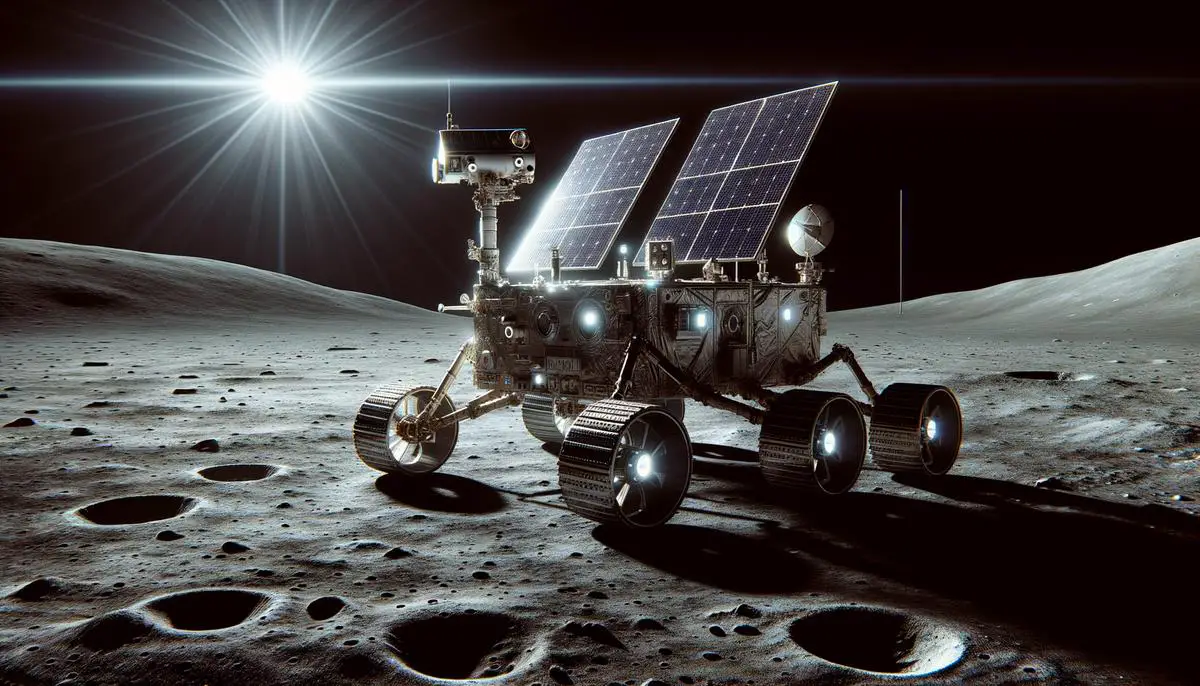
In conclusion, the struggle against lunar dust encapsulates the broader challenge of conducting missions on the moon. It underscores a critical lesson: success in lunar endeavors hinges on our ability to adapt to and mitigate the harsh conditions of an alien environment. This principle of adaptability, demonstrated through our ongoing efforts to protect astronauts and technology from lunar dust, is pivotal for future missions, ensuring that we can continue to push the boundaries of what is possible in space exploration.
- Cain JR. Lunar dust: The hazard and astronaut exposure risks. Astrobiology. 2010;10(10):1093-1100.
- Paige DA, Foote MC, Greenhagen BT, et al. The Lunar Reconnaissance Orbiter Diviner Lunar Radiometer Experiment. Space Sci Rev. 2010;150(1-4):125-160.
- Fong T, Bualat M, Deans M, et al. Field testing of utility robots for lunar surface operations. In: AIAA SPACE 2008 Conference & Exposition. 2008.
- NASA. The Artemis Accords: Principles for a safe, peaceful, and prosperous future. 2020.
- Heiken G, Vaniman D, French BM, eds. Lunar Sourcebook: A User's Guide to the Moon. CUP Archive; 1991.
- Maurer RH, Fraeman ME, Martin MN, et al. Harsh Environments: Space Radiation. Johns Hopkins APL Technical Digest. 2008;28(1):17-29.