The Birth of the Universe
Around 13.8 billion years ago, the universe began with the Big Bang. This event marked the start of cosmic inflation, where the universe expanded faster than the speed of light, growing from smaller than a proton to golf ball-sized within fractions of a second.
As the universe cooled, it became a primordial soup of particles, including neutrons, protons, electrons, anti-electrons, and neutrinos. Within the first three minutes, light elements like hydrogen, helium, and a small amount of lithium formed, becoming the building blocks for stars and galaxies.
For about 380,000 years, the universe remained opaque. As it cooled further, electrons combined with protons and neutrons to form neutral atoms, allowing light to travel freely. This ancient light, known as the cosmic microwave background (CMB), is still detectable today.
Around 400 million years after the Big Bang, the first stars and galaxies began to form as gas clouds collapsed under gravity. Dark matter, a hypothetical form of matter that doesn't interact with light, played a crucial role in this process by providing a gravitational scaffold for galaxy formation.
Scientists use detailed simulations to understand this cosmic evolution, matching models with CMB data and other observations to confirm our understanding of the universe's early structures and ongoing expansion.
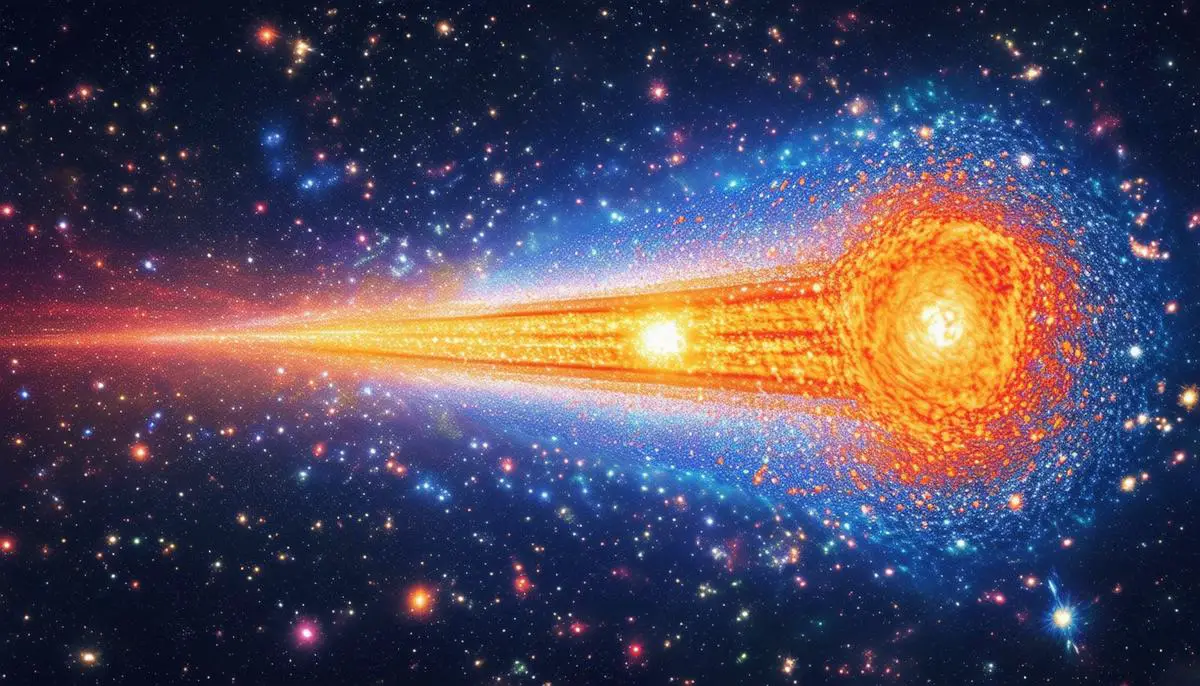
Evidence Supporting the Big Bang Theory
Edwin Hubble's work in the 1920s provided compelling evidence for the Big Bang Theory. His observations revealed that galaxies are moving away from us, with their speed proportional to their distance from Earth. This phenomenon, known as Hubble's Law, indicates a uniform expansion of the universe in all directions.1
The cosmic microwave background (CMB) radiation, discovered by Arno Penzias and Robert Wilson in 1965, offers another key piece of evidence. The CMB provides a snapshot of the universe about 380,000 years after its inception. Detailed maps of the CMB from missions like NASA's COBE and the ESA's Planck satellite show tiny temperature fluctuations corresponding to early density variations in the universe.
Key Evidence for the Big Bang Theory:
- Hubble's Law and galactic redshift
- Cosmic Microwave Background radiation
- Light element abundances
- Large-scale structure of the universe
The relative abundances of light elements in the cosmos also support the Big Bang Theory. During the first few minutes of the universe's existence, conditions allowed for the formation of hydrogen, helium, and traces of lithium through a process called Big Bang nucleosynthesis. The observed ratios of these elements align well with theoretical predictions.
Advanced simulations and theoretical models consistently verify these observations. Scientists use supercomputers to simulate the universe's evolution based on Big Bang conditions, producing results that match our detailed observations of galaxies, cosmic structures, and the CMB.
The convergence of data from Hubble's Law, CMB properties, and light element abundances creates a solid framework supporting the Big Bang Theory. This evidence aligns with theoretical predictions and withstands rigorous testing, reinforcing our understanding of the universe's origins and evolution.
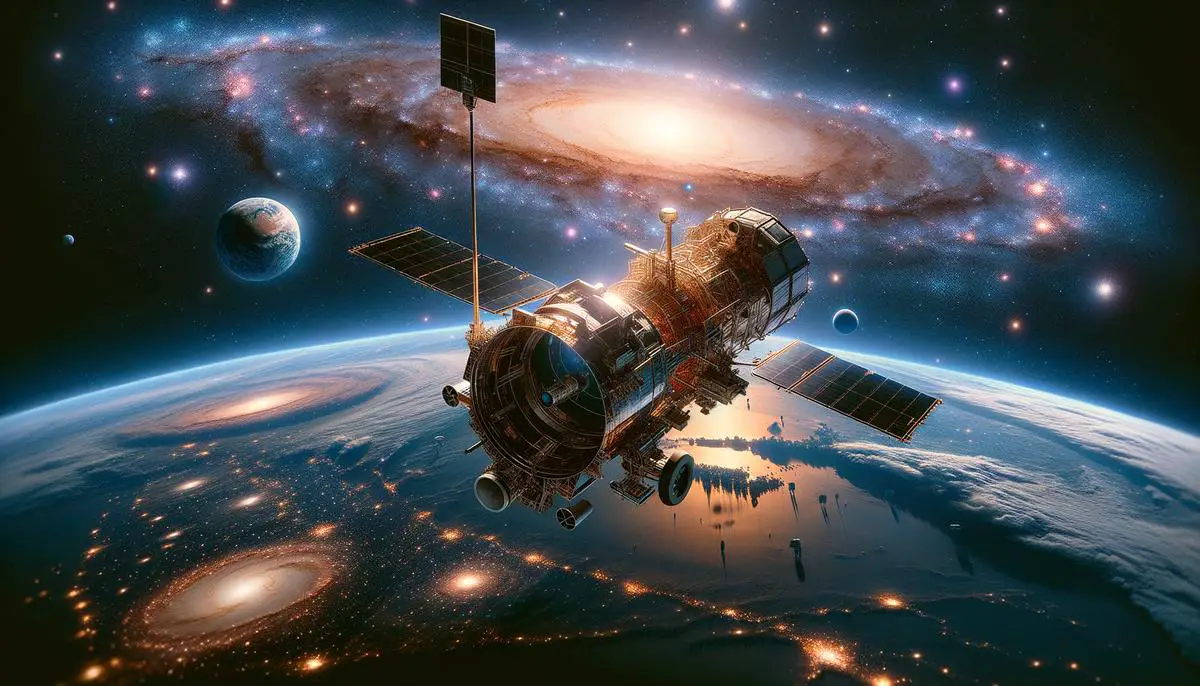
Alternative Theories and Challenges
While the Big Bang Theory is widely accepted, alternative models have been proposed. The theory of eternal inflation suggests that rapid expansion continues in various regions of space, with our observable universe being one "bubble" in a vast multiverse. The oscillating universe or cyclic model proposes that the universe undergoes continuous cycles of expansion and contraction.
Challenges to the Big Bang Theory:
- The horizon problem
- The flatness problem
- The Hubble tension
The Big Bang Theory faces some challenges, including the horizon problem and the flatness problem. The horizon problem questions how regions of the universe that are too far apart to have ever been in contact can have the same temperature. The flatness problem addresses the improbable precision required in initial conditions for the universe to be geometrically flat.
The theory of cosmic inflation helps address these issues by proposing a period of rapid expansion in the early universe. However, some challenges remain, such as the Hubble tension—discrepancies in the measured rate of expansion of the universe between early and late universe observations.2
"Each unexplained phenomenon presents an opportunity to broaden our understanding of the cosmos, guiding us towards uncovering the true nature of our vast and complex universe."
These challenges and alternative theories drive the field forward, encouraging the development of more sophisticated models and precise observations. As we explore deeper, our current theories may need refinement or even complete revision.
The Expanding Universe
The concept of the expanding universe, first supported by Edwin Hubble's observations in the 1920s, reveals that galaxies are moving away from each other as space itself stretches. This expansion is uniform throughout the observable universe.
Dark energy, a mysterious force discovered in the late 20th century, appears to drive the accelerated expansion of the universe. Constituting about 68% of the total energy density of the universe, dark energy counteracts the effects of gravity. Its exact nature remains one of the most profound mysteries in cosmology.
The discovery that the universe's expansion is accelerating was a paradigm shift in our understanding. This acceleration was first observed through studies of distant Type Ia supernovae, which act as cosmic mile markers. Several theories attempt to explain dark energy, including the cosmological constant hypothesis and the concept of quintessence.
Potential Scenarios for the Future of the Universe:
Scenario | Description |
---|---|
The "Big Freeze" or "Heat Death" | The universe continues to expand and cool, eventually leading to a dark, lifeless state. |
The "Big Rip" | If dark energy increases in strength, it could ultimately tear apart all structures in the universe. |
The "Big Crunch" | A reversal of expansion could lead to the universe collapsing back into a dense state. |
A cyclical universe | Alternating Big Bang and Big Crunch events create an eternal cycle of birth, death, and rebirth. |
The ultimate fate of the universe depends on factors such as the true nature of dark energy and the universe's geometry. As we continue to study the expanding universe, we gain insights into both the scientific and philosophical aspects of our cosmic existence, driven by our curiosity to explore the unknown.
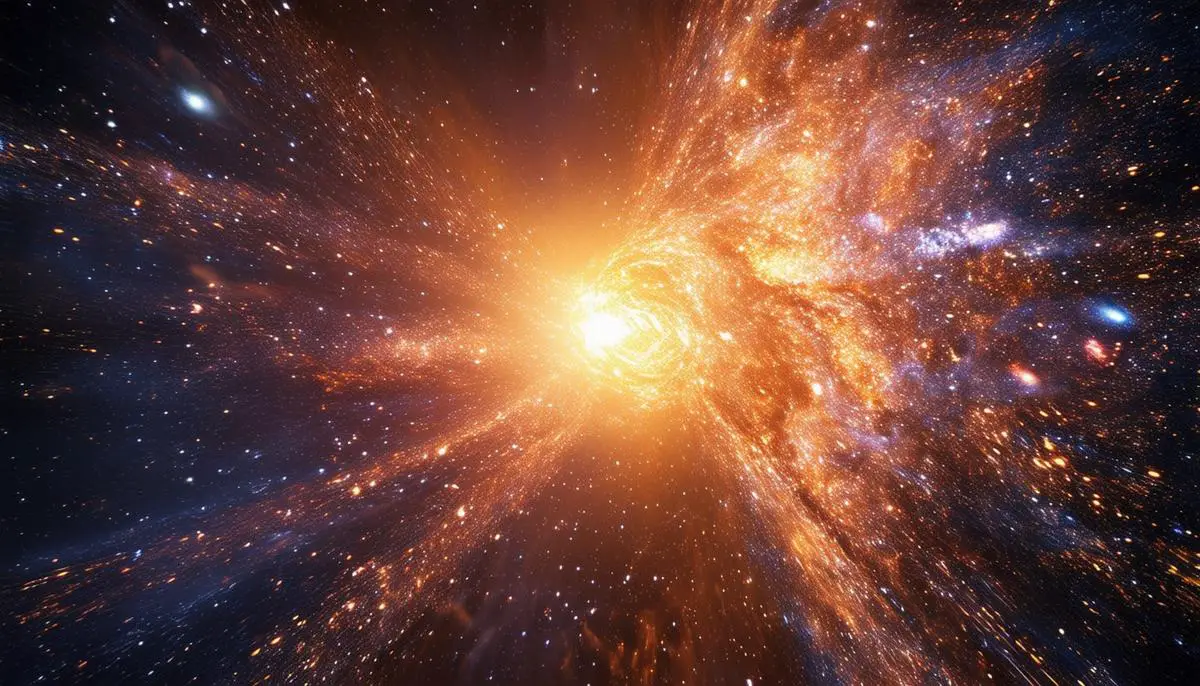
Gravitational Waves and Cosmic Backgrounds
The detection of gravitational waves marks a significant advancement in our understanding of the universe's early moments, providing a novel way to explore the cosmos. Gravitational waves, ripples in spacetime predicted by Einstein's general theory of relativity, are generated by some of the most energetic processes in the universe, such as merging black holes and neutron stars. They hold valuable clues about the universe's infancy, moments after the Big Bang.
First observed in 2016 by LIGO (Laser Interferometer Gravitational-Wave Observatory), these waves have since been detected multiple times, confirming their existence. The discovery earned the 2017 Nobel Prize in Physics, underscoring its importance. Gravitational waves offer a unique perspective about the universe's birth and evolution that light itself cannot provide.
The significance of gravitational waves in studying the early universe is multifaceted:
- During the first fraction of a second after the Big Bang, the universe underwent inflation, a period of rapid expansion.
- This stretching would have generated gravitational waves, carrying information about the early universe that is otherwise inaccessible.
- These primordial gravitational waves could offer insights into the conditions and physics that dominated during that fleeting moment of rapid expansion.
These waves could help verify different models of inflation — whether it was a single, sudden burst or involved multiple, complex phases. If detected, the polarization patterns in these waves could potentially confirm theories of cosmic inflation. Efforts continue, using highly sensitive instruments such as the European Space Agency's Planck satellite and the upcoming LISA (Laser Interferometer Space Antenna) mission, to detect the faint imprints of primordial gravitational waves.
Alongside gravitational waves, the cosmic microwave background (CMB) radiation provides another crucial window into the early universe. The CMB is the afterglow of the Big Bang, offering a snapshot of the universe when it was just 380,000 years old. This relic radiation is remarkably uniform, yet contains tiny fluctuations that correspond to regions of varying density in the early universe. These fluctuations eventually became the seeds of all the structures we see today, from galaxies to clusters of galaxies.
The discovery of the CMB in 1965 by Arno Penzias and Robert Wilson was a watershed moment, confirming the Big Bang Theory and earning them the Nobel Prize. Detailed observations of the CMB, especially from missions like COBE, WMAP, and Planck, have provided a wealth of data. These observations have mapped the temperature fluctuations across the sky with increasing precision, offering clues about the universe's composition and its rate of expansion.
The CMB also informs our understanding of dark matter and dark energy. By analyzing the CMB's temperature and polarization data, scientists can infer the amounts of dark matter and dark energy, providing a coherent picture of the universe's current composition and how it has evolved over billions of years.
While the CMB and gravitational waves are distinct phenomena, they complement each other in the grand story of cosmic evolution. Gravitational waves offer a direct probe of the universe's earliest moments, potentially confirming theories of inflation. In contrast, the CMB provides a detailed view of the universe's state a few hundred thousand years later, helping refine our models of how matter and energy have shaped the cosmos.
Ongoing research in these fields continues to push the boundaries of our knowledge. Instruments like upgraded gravitational wave detectors and next-generation CMB observatories promise to yield even more data, bringing us closer to answering fundamental questions about the universe's origins and its fate.
Modern Observations and Future Research
Modern observations and future research in cosmology continue to refine our knowledge of the cosmos. A pivotal development has been the launch and operation of the James Webb Space Telescope (JWST). The JWST's ability to observe in the infrared spectrum allows it to peer back in time to the formation of the first galaxies, providing a clearer picture of the universe's infancy.
One notable achievement of the JWST was the discovery of high-redshift galaxies, such as UNCOVER-z13 and UNCOVER-z12, situated mere hundreds of millions of years after the Big Bang. Unlike other high-redshift galaxies, which appear point-like and small, the UNCOVER galaxies exhibit structure and are relatively large, suggesting diversity in galaxy formation even in the universe's earliest stages.1
Other observatories continue to play crucial roles in advancing cosmology:
- The European Space Agency's Planck satellite, with its detailed mapping of the CMB, has refined our understanding of the universe's age, composition, and fundamental parameters.
- Planck's data has shown a universe that is surprisingly flat, aligning closely with the predictions of the inflationary model while also prompting questions about the exact nature of dark energy and dark matter.
The study of Type Ia supernovae remains vital for understanding cosmic expansion. Recent findings indicate a discrepancy, known as the Hubble tension, between expansion rates inferred from supernovae data and those derived from the CMB. This tension suggests that there might be aspects of cosmology that remain undiscovered or inadequately explained by current models.
The search for dark matter continues, employing a variety of methods including:
- Direct detection experiments
- High-energy particle collisions at the Large Hadron Collider
- Indirect observations through cosmic rays and gamma rays
Identifying dark matter remains one of the most pressing questions in cosmology, as it constitutes roughly 23% of the universe's mass-energy content.
Future research also focuses extensively on dark energy, which drives the accelerated expansion of the universe. Projects like the Dark Energy Survey (DES) and the upcoming Euclid mission aim to map dark energy's influence over time and space. Gravitational lensing provides another tool to study dark energy's effects and refine cosmic distance scales.
The Laser Interferometer Space Antenna (LISA), slated for launch in the 2030s, promises to expand our gravitational wave observatory capabilities into space. By detecting lower-frequency gravitational waves, LISA will observe events such as the mergers of supermassive black holes and potentially even primordial gravitational waves from the Big Bang.
"The synergy between various observational methods creates a multi-faceted approach to cosmology. These diverse methodologies enable scientists to cross-validate findings, test theoretical models, and explore previously inaccessible regions of the universe."
In addition to observational advancements, theoretical developments continue to push the boundaries of our knowledge. Models incorporating quantum mechanics, general relativity, and particle physics aim to build a coherent, unified description of the cosmos, furthering our comprehension of phenomena from black holes to the Big Bang itself.
As cutting-edge tools uncover new layers of complexity in the cosmos, they draw us closer to answering some of the most profound questions in science, continuing the age-old human quest to grasp the mysteries of our universe.
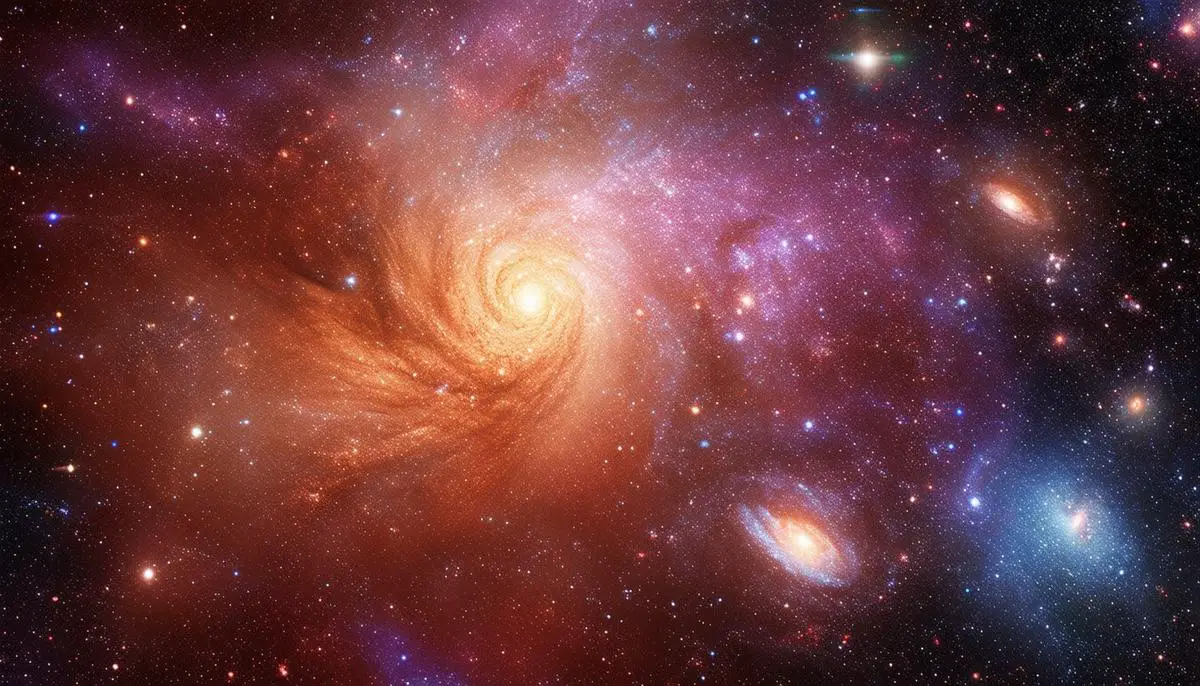
In sum, the universe's expansion is a testament to the intricate nature of the cosmos, continuously inviting us to unravel its mysteries and comprehend our place within this grand, unfolding story. As we study an ever-expanding universe, the quest for understanding becomes both a scientific journey and a philosophical odyssey, driven by our curiosity to explore the unknown.