Foundations of the Cosmic Distance Ladder
Measuring cosmic distances is challenging. To determine how far away stars, galaxies, and other celestial bodies are, astronomers use the cosmic distance ladder. Each step, or "rung," involves different techniques that build upon one another, allowing scientists to reach greater distances with each successive step.
The cosmic distance ladder starts with parallax, the oldest method for measuring distances to stars within a few thousand light-years. Just like when you look at an object with one eye closed and then the other, stars appear to shift against a distant backdrop as Earth orbits the Sun. The amount of apparent movement gives us the distance.
Cepheid variables offer the celestial equivalent of a lighthouse beacon for gauging farther distances. These stars pulse in brightness at a rate that directly relates to their intrinsic luminosity. With a known brightness, astronomers can deduce their distance just by observing how they sparkle. Cepheids let us measure up to 100 million light-years or so.
Type Ia supernovae are stellar explosions that burst forth with consistent brightness, becoming handy markers for distance calculation. Because they shine so brightly, they're visible up to about 10 billion light-years away. Supernovae essentially continue where Cepheids stop, letting astronomers map distances across vast swaths of the universe.
Redshift, the cosmic version of a Doppler effect, allows us to infer distances using Hubble's law. As galaxies move away from us, their light stretches into longer, redder wavelengths. This method has spotted galaxies as far back as 13 billion light-years.
Despite its utility, the cosmic distance ladder isn't flawless. Each step introduces errors, which can add up as distances grow. Recent technology and data from devices like the Gaia spacecraft aim to narrow down these uncertainties.
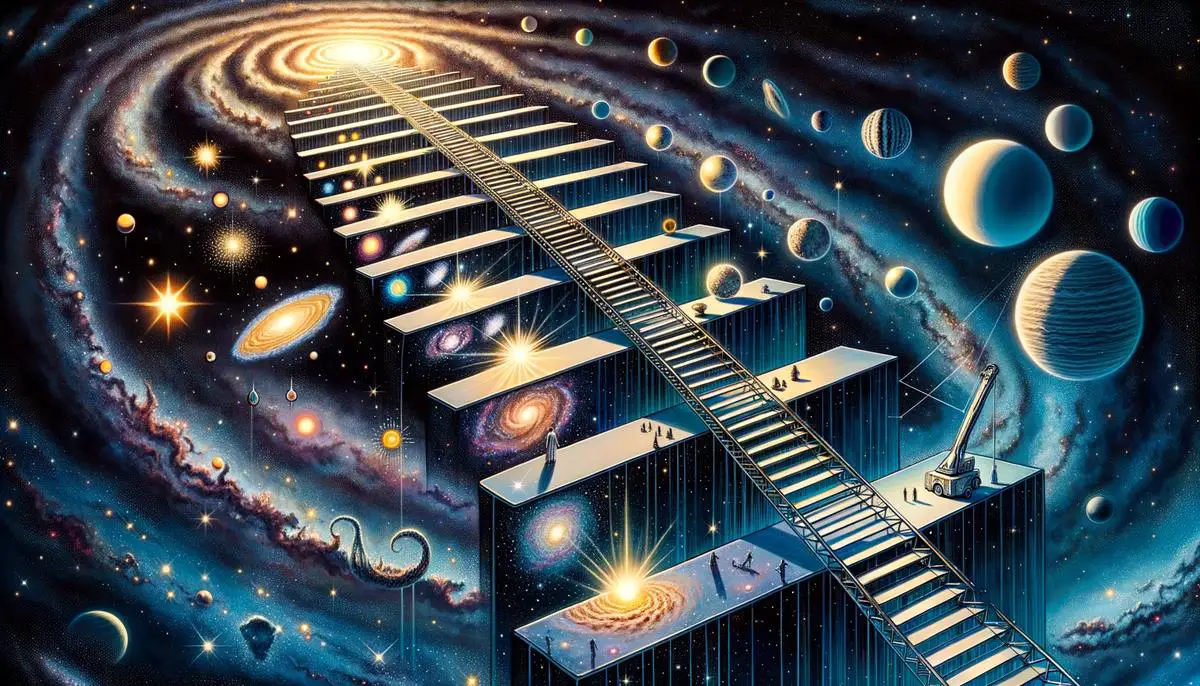
Parallax and Nearby Stars
Parallax serves as the foundation of the cosmic distance ladder. When Earth revolves around the Sun, nearby stars seem to shift position when viewed against the vast backdrop of faraway stars. This apparent shift, or parallax angle, offers the key to estimating how far away those stars are.
Due to the immense distances involved, these shifts are tiny, often requiring careful observations over a six-month period as the Earth moves from one side of its orbit to the other. This method is effective but limited to stars within a couple of thousand light-years.
Space-based observatories like Hipparcos and Gaia revolutionized parallax measurements. Launched by the European Space Agency, Hipparcos mapped over 100,000 stars with unprecedented precision in the late 20th century. Since its launch in 2013, Gaia has been charting over a billion stars with astoundingly fine detail, reducing the errors in measurement significantly.
"With the advent of huge telescopes like the Extremely Large Telescope, we will be able to conduct studies of nearby galaxies with unprecedented accuracy, making it vital that we already know precisely how far away they are."
The impact of Gaia on the cosmic distance ladder is significant. Its refined data provides the most solid foundation yet for further rungs. By tightening the accuracy of the initial parallax measurements, Gaia helps ensure that the subsequent steps involving Cepheids and Type Ia supernovae remain more aligned with reality.
As technology advances and new missions line up on the horizon, the aspiration is to push even beyond current boundaries, sharpening our cosmic map and bringing the universe ever closer to our understanding.
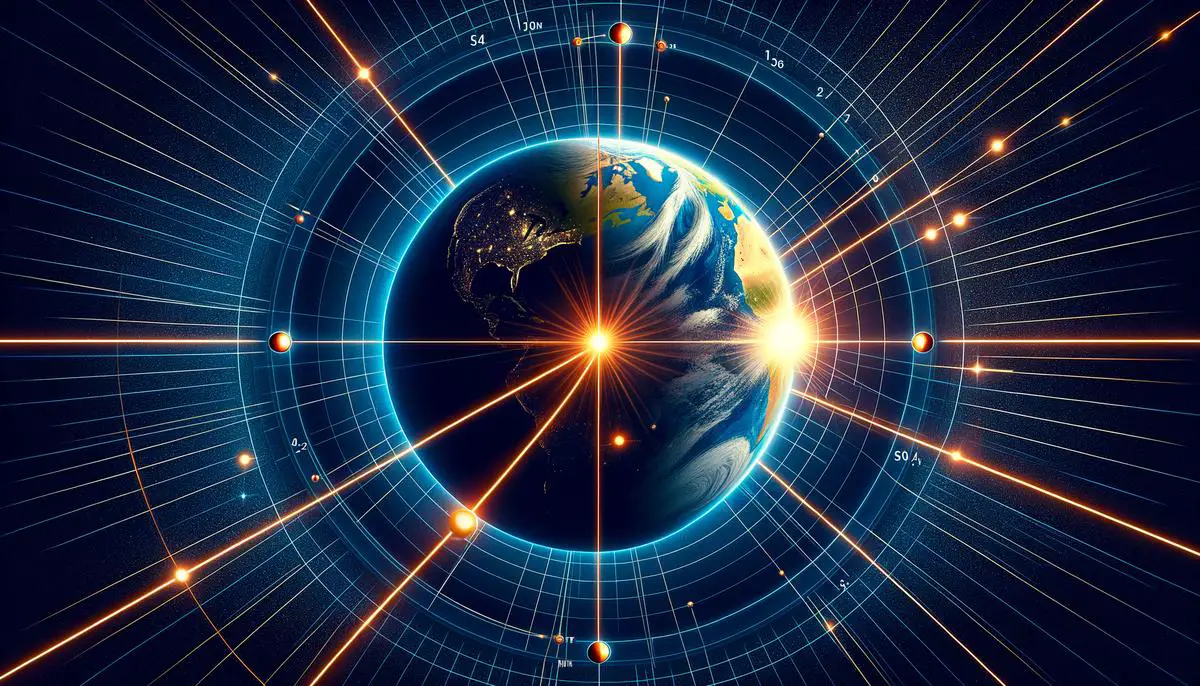
Cepheid Variables and the Period-Luminosity Relationship
Cepheid variables extend the cosmic distance ladder into more distant territories. These stars vary in brightness with such reliability that they offer one of the most dependable measures in astronomy.
The magic of Cepheid variables lies in their pulsations. These stars brighten and dim at intervals that relate closely to their intrinsic luminosity. Henrietta Swan Leavitt discovered this vital relationship known as the Period-Luminosity Relationship. By observing the period of a Cepheid's brightness changes, astronomers can accurately ascertain its true brightness. Once the intrinsic brightness is known, determining its distance is a simple matter of comparing this brightness to the star's apparent brightness as seen from Earth.
This method allows astronomers to measure distances millions of light-years away, where parallax alone could never reach. Each Cepheid we study helps us to pinpoint other galaxies, effectively turning the universe into a mapped grid.
The precision offered by the Period-Luminosity Relationship is pivotal—not only for measuring galaxies but for calibrating other distance measurement techniques. When we know the distance to galaxies hosting Cepheids, we can tune the accuracy of other rungs of the cosmic distance ladder, such as Type Ia supernovae.
The advancement of space observatories continues to refine and expand our understanding of Cepheid variables. Instruments like the Hubble Space Telescope and the Gaia mission have dramatically increased the number of known Cepheid stars and the accuracy of their data.
Type Ia Supernovae as Standard Candles
Type Ia supernovae occur in binary star systems when a white dwarf accretes enough material from its companion star, triggering a colossal explosion. Remarkably, the peak brightness of such supernovae is always nearly identical. This makes them reliable "standard candles," ideal for gauging distances across the cosmos.
By knowing their intrinsic brightness, Type Ia supernovae serve as cosmic lighthouses, beaming information about their home galaxies' distances back to Earth. Once astronomers measure the light's apparent brightness, they can compare it to the supernova's known luminosity, allowing distance calculations that extend to around 10 billion light-years.
For cosmologists, these supernovae have been pivotal in studying the universe's expansion. The relationship between a galaxy's distance and its recession speed, as established by Edwin Hubble—known as the Hubble Constant—was fine-tuned through measurements using Type Ia supernovae.
However, this task isn't without its challenges. Despite their utility, the uniform brightness of Type Ia supernovae isn't absolute. Small variations and other influencing cosmic phenomena necessitate careful standardization and calibration. This is where cross-validation with other rung methods, like Cepheids, proves invaluable, ensuring the precision needed at these astronomical distances.
Modern observatories continue to refine these calibrations, unraveling the layers of cosmic history buried within these stellar explosions. As telescopes become more powerful and data more precise, Type Ia supernovae continue to illuminate not just the comprehensive cosmic map but the mysteries of the universe, from its very birth to its speculated endless expansion.
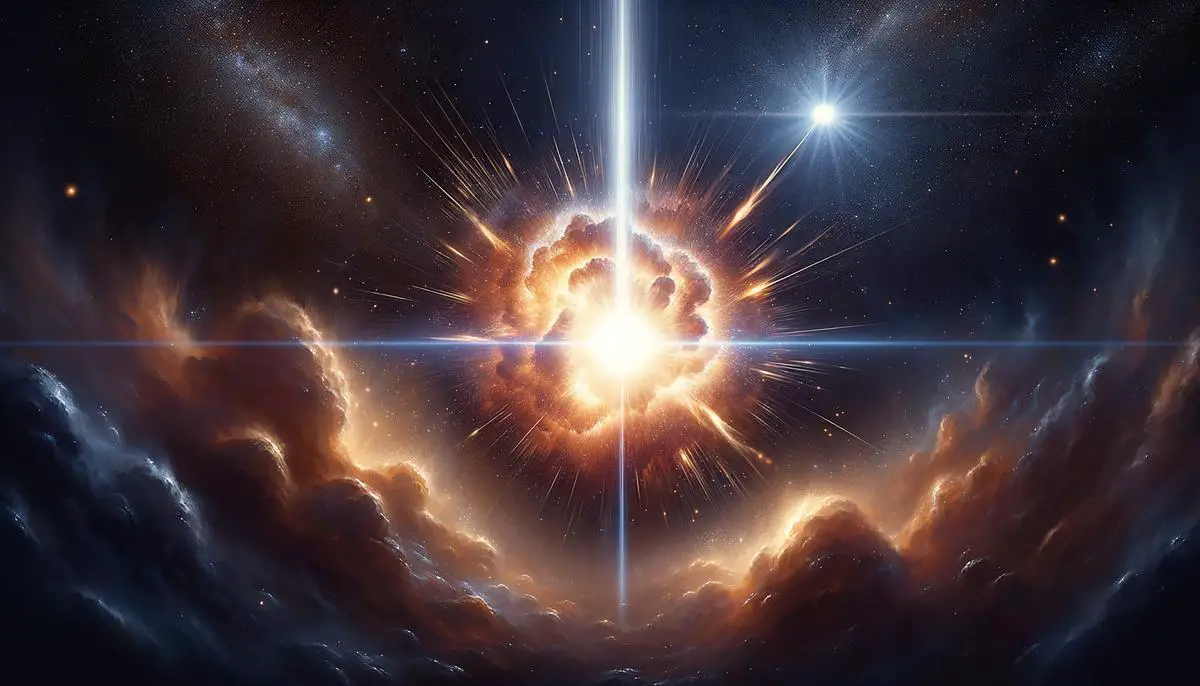
Challenges and Cosmic Tension
As we ascend the cosmic distance ladder, one of the most difficult tasks is dealing with the inherent challenges and uncertainties that persist at each step. This issue often introduces what we term as cosmic tension—a phenomenon where various distance measurement techniques provide conflicting results.
Each method in the cosmic ladder comes with its own set of potential errors:
- Parallax becomes less precise at greater distances due to minuscule angle shifts
- Cepheid variables can be obscured by interstellar dust, leading to miscalculations
- Type Ia supernovae require careful standardization to account for variations
These accumulating challenges manifest as cosmic tension—a term that encompasses the discrepancies observed when different methodologies yield divergent results for the same cosmic distances. This tension is most apparent in the measurements of the Hubble Constant, which describes the universe's expansion rate.
For astrophysicists, resolving cosmic tension is an ongoing mission. With each refinement in technological capability, such as newer data from the Gaia spacecraft or advances in space telescope technology, scientists aim to tighten these uncertainties and bring our picture of the universe into clearer focus.
In the quest for precision, balancing cosmic tension involves developing novel methods, enhancing current models, and welcoming continual improvements in measurement technologies. Such efforts promise not just better accuracy, but open up deeper insights into cosmic phenomena like dark energy, allowing science to edge ever closer to understanding the true scale and evolution of the cosmos.
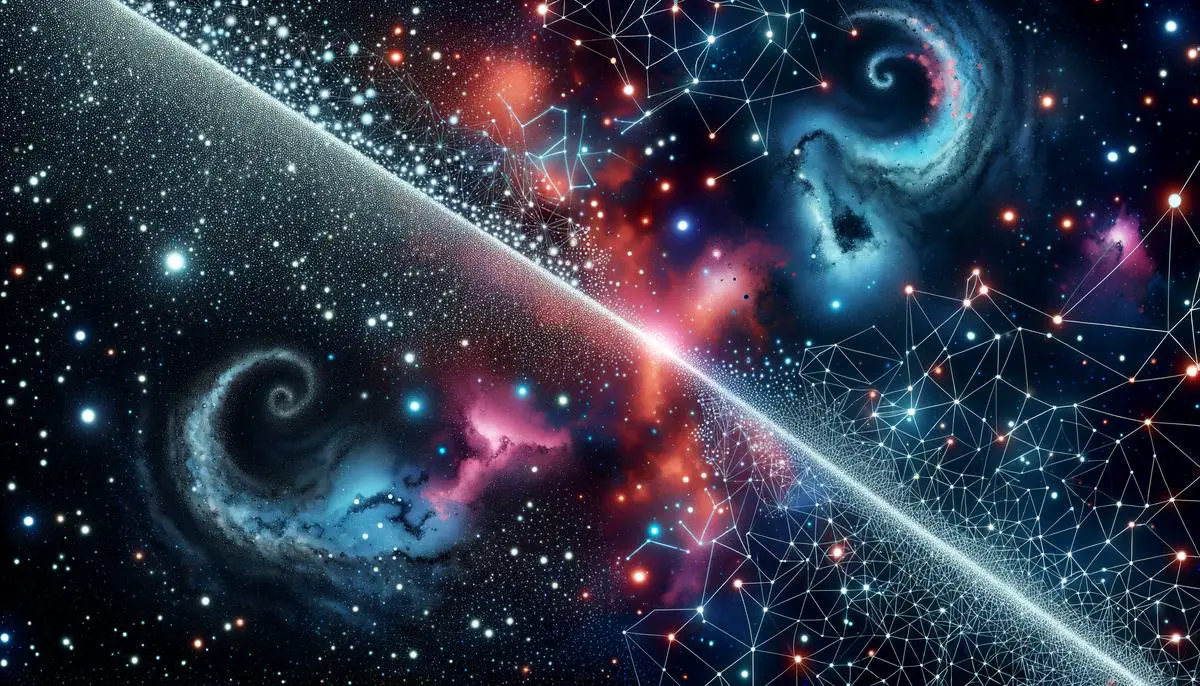
In the vast expanse of the universe, the cosmic distance ladder stands as a testament to human curiosity and ingenuity. It is through this intricate framework that astronomers piece together the vast distances that separate us from the stars. Each method, from parallax to Type Ia supernovae, contributes to a deeper understanding of our universe's scale and expansion. As technology advances, so too does our ability to refine these measurements, bringing us ever closer to unraveling the mysteries of the cosmos.
- Riess AG, Yuan W, Casertano S, et al. Cosmic distances calibrated to 1% precision with Gaia EDR3 parallaxes and Hubble Space Telescope photometry of 75 Milky Way Cepheids confirm tension with LambdaCDM. arXiv preprint. 2020.
- Koberlein B. Measuring cosmic expansion continues to be a challenge. Universe Today. January 3, 2021.