Normal Matter vs. Dark Matter
Normal matter, comprising less than 5% of the universe, is made of protons, neutrons, and electrons. It's visible, tangible, and forms everything from planets to living beings. Dark matter, accounting for about 27% of the universe, doesn't interact with light and is only detectable through its gravitational effects.
Normal matter's composition is well understood: atoms with nuclei orbited by electrons. Dark matter's makeup remains hypothetical, with candidates like WIMPs (weakly interacting massive particles) or axions proposed.
While normal matter is easily observable through various methods, dark matter's presence is inferred through its gravitational influence on galaxies and cosmic structures. Together, they shape the universe's layout, with normal matter forming visible celestial bodies and dark matter providing the gravitational framework that holds galaxies together.
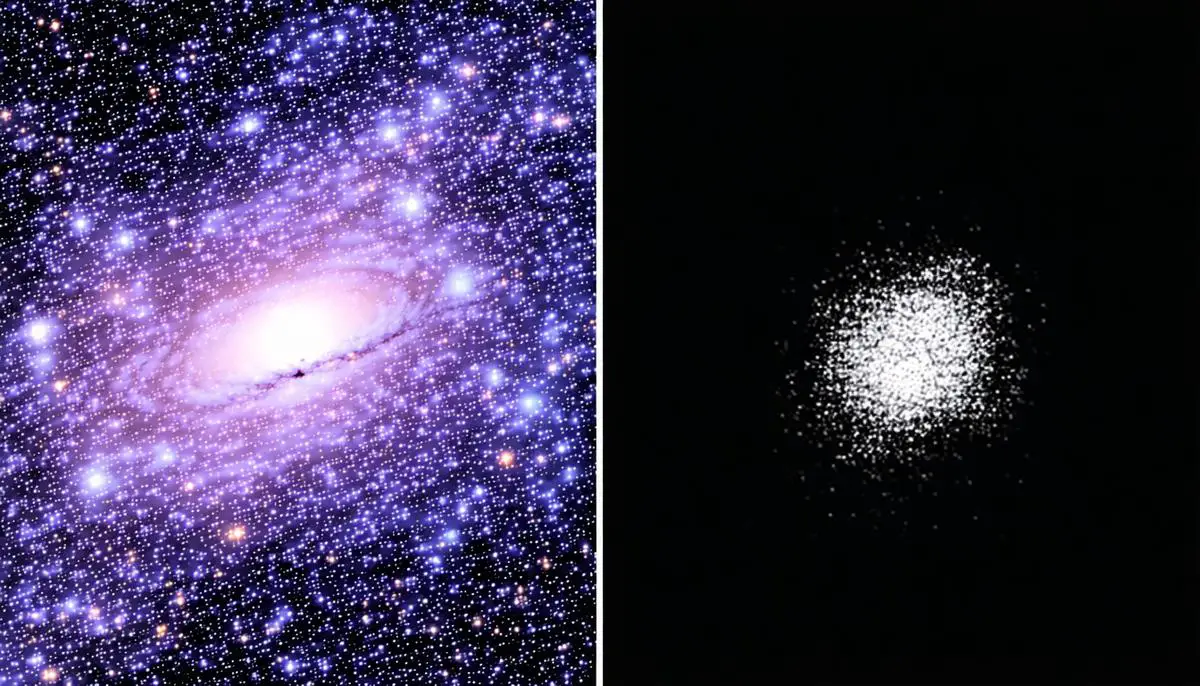
The Evidence for Dark Matter
Galaxy rotation curves provide compelling evidence for dark matter. Stars at the outer edges of galaxies move faster than expected based on visible mass alone, suggesting additional gravitational influence from unseen matter.
Gravitational lensing, where light from distant objects is bent around massive cosmic structures, reveals more mass than is visibly present. This phenomenon supports the existence of dark matter acting as invisible scaffolding for the universe.
The cosmic microwave background (CMB) radiation, an echo of the early universe, exhibits patterns indicating dark matter's gravitational influence even in the universe's infancy.
Historical Context
- 1930s: Fritz Zwicky introduces the concept of dark matter through galaxy cluster observations
- 1970s: Vera Rubin's studies on galaxy rotation provide further support
While direct detection remains elusive, these phenomena collectively build a strong case for dark matter's existence and its crucial role in cosmic structure.
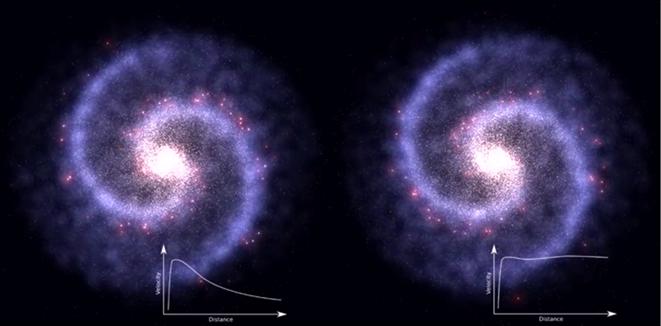
Theories and Candidates for Dark Matter
Several candidates for dark matter have been proposed:
- Weakly Interacting Massive Particles (WIMPs): These hypothetical particles interact minimally with normal matter and fit well within existing particle physics frameworks.
- Axions: Low-mass particles that could potentially solve multiple cosmic puzzles, including the strong CP problem in quantum physics.
- Sterile neutrinos: Even less interactive than known neutrinos, these particles might only reveal themselves through gravitational effects and specific nuclear reactions.
Detecting these elusive particles requires innovative approaches. The Large Hadron Collider attempts to recreate early universe conditions to possibly produce dark matter particles. Underground detectors like XENON1T use noble gases to capture rare WIMP interactions. Axion detectors employ various techniques, from powerful magnets to resonant antennae, to search for these particles' signatures.
Each experiment, successful or not, refines our understanding and narrows the search parameters for dark matter. This ongoing pursuit not only seeks to identify dark matter but also aims to deepen our comprehension of the universe's fundamental structure.
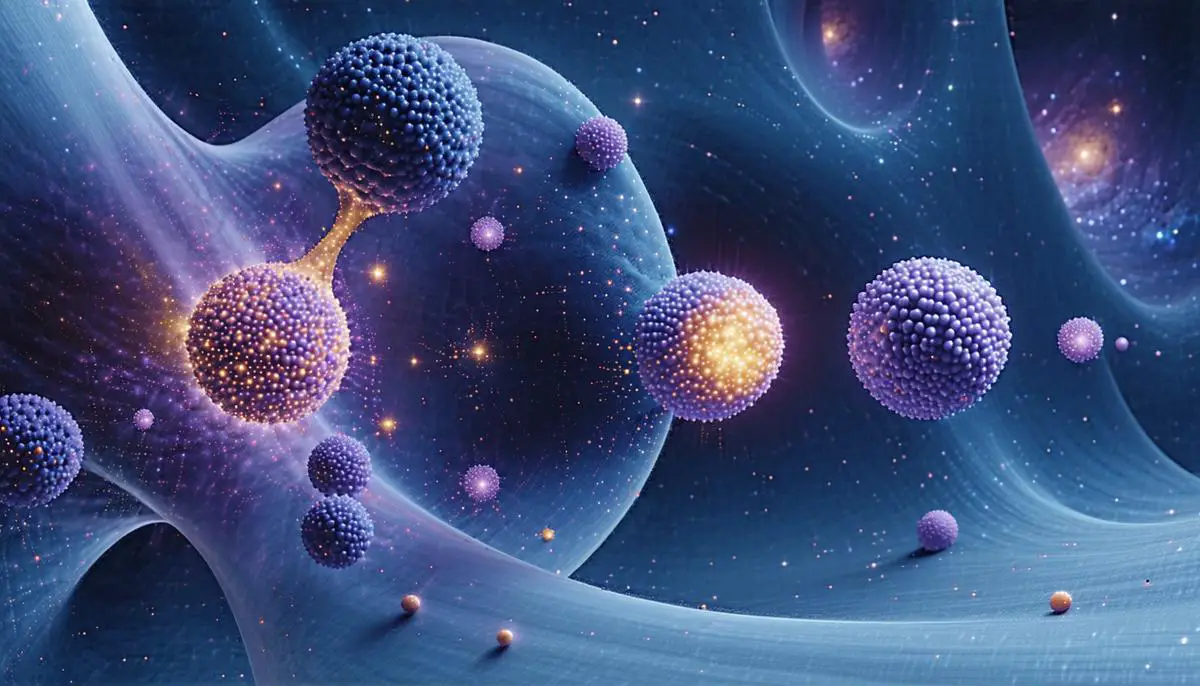
Dark Matter's Role in Cosmic Structure
Dark matter serves as the cosmic framework, guiding the formation and evolution of large-scale structures in the universe. Its gravitational influence shapes the cosmic web—a vast network of galaxy clusters and filaments interspersed with voids.
In the early universe, dark matter's gravity amplified tiny fluctuations in matter distribution, leading to the formation of stars and galaxies. It continues to play a crucial role in maintaining galactic integrity, preventing stars from escaping their host galaxies despite their high rotational speeds.
Dark matter's ongoing influence shepherds galaxies through space, facilitating collisions and mergers that drive galactic evolution. These interactions shape galaxy size, star formation rates, and stellar populations.
Advanced observatories like the Rubin Observatory and Euclid Mission are mapping dark matter's distribution by studying gravitational lensing and galaxy movements. These observations provide insights into cosmic history and structure formation, while also offering clues about dark energy and the universe's expansion.
By studying dark matter, scientists aim to unravel the complexities of cosmic evolution and better understand the place of galaxies like our Milky Way in the grand cosmic narrative.
Dark Energy and Its Relationship with Dark Matter
Dark energy, comprising about 68% of the universe, is the mysterious force driving the accelerated expansion of the cosmos. Unlike the concentrated effects of dark matter, dark energy exerts a uniform influence across space, pushing galaxies apart at an increasing rate.
The relationship between dark matter and dark energy is complex. Dark matter's gravitational pull binds cosmic structures together, while dark energy drives them apart. This interplay shapes the universe's evolution and potential future.
The discovery of accelerated cosmic expansion challenged previous assumptions about the universe's fate. If dark energy continues to dominate, it could lead to a "Big Freeze," where expansion continues indefinitely. Conversely, if dark matter's influence increases, it might result in a "Big Crunch," where the universe collapses back on itself.
Missions like Euclid aim to study how galaxies have evolved under the influence of both dark matter and dark energy, offering insights into their fundamental nature and the universe's destiny.
"The whole purpose of Euclid is really to put those two together to understand the nature of dark matter and dark energy and how they're coupled in the universe," Carole Mundell, ESA director of science.
Understanding the balance between these cosmic forces is crucial for unraveling the universe's past and predicting its future, driving ongoing research and technological advancements in cosmology.
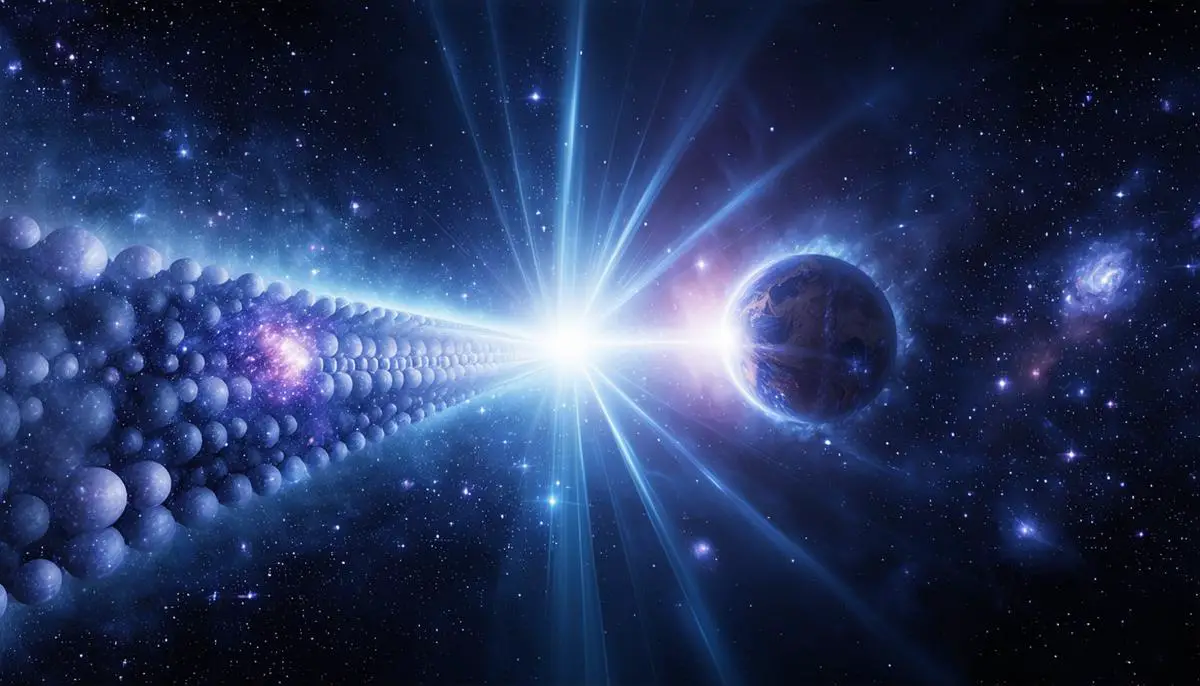
As we continue to explore the cosmos, dark matter remains a central mystery in our understanding of the universe. Its gravitational influence shapes cosmic structures and galactic dynamics, holding keys to unlocking the secrets of our celestial home. Ongoing research promises to deepen our comprehension of the universe's grand design, propelling us toward new frontiers in cosmological knowledge.
- Zwicky F. Die Rotverschiebung von extragalaktischen Nebeln. Helv Phys Acta. 1933;6:110-127.
- Rubin VC, Ford WK Jr. Rotation of the Andromeda Nebula from a Spectroscopic Survey of Emission Regions. Astrophys J. 1970;159:379.
- Planck Collaboration. Planck 2018 results. VI. Cosmological parameters. Astron Astrophys. 2020;641:A6.
- Aprile E, Aalbers J, Agostini F, et al. Dark Matter Search Results from a One Ton-Year Exposure of XENON1T. Phys Rev Lett. 2018;121:111302.
- Ade PAR, Aghanim N, Arnaud M, et al. Planck 2015 results. XIII. Cosmological parameters. Astron Astrophys. 2016;594:A13.