Quantum Mechanics and Microscopic Scales
Quantum mechanics governs the behavior of matter and energy at the microscopic level, challenging our classical understanding of physics. At its core is Planck's constant, which sets a fundamental limit to how precisely we can measure certain properties of particles.
When dealing with electric forces at microscopic scales, we encounter Coulomb's law, describing the interactions between charged particles. These forces are significantly stronger than gravity at this level.
Quantum mechanics redefines our understanding of size and position. Electrons and protons exist in probabilistic orbits rather than fixed locations. The 'microscopic' scale in quantum mechanics is measured in Ångströms, or billionths of meters.
Light also plays a crucial role in quantum mechanics. Beyond visible light, there are energies that can affect electrons or break molecular bonds, illustrating the influence of quantum mechanics on atomic interactions.
Occasionally, quantum effects manifest on a larger scale, as seen in superconductivity and superfluidity. These phenomena demonstrate quantum principles at work in macroscopic systems, allowing materials to exhibit zero electrical resistance or flow without viscosity.
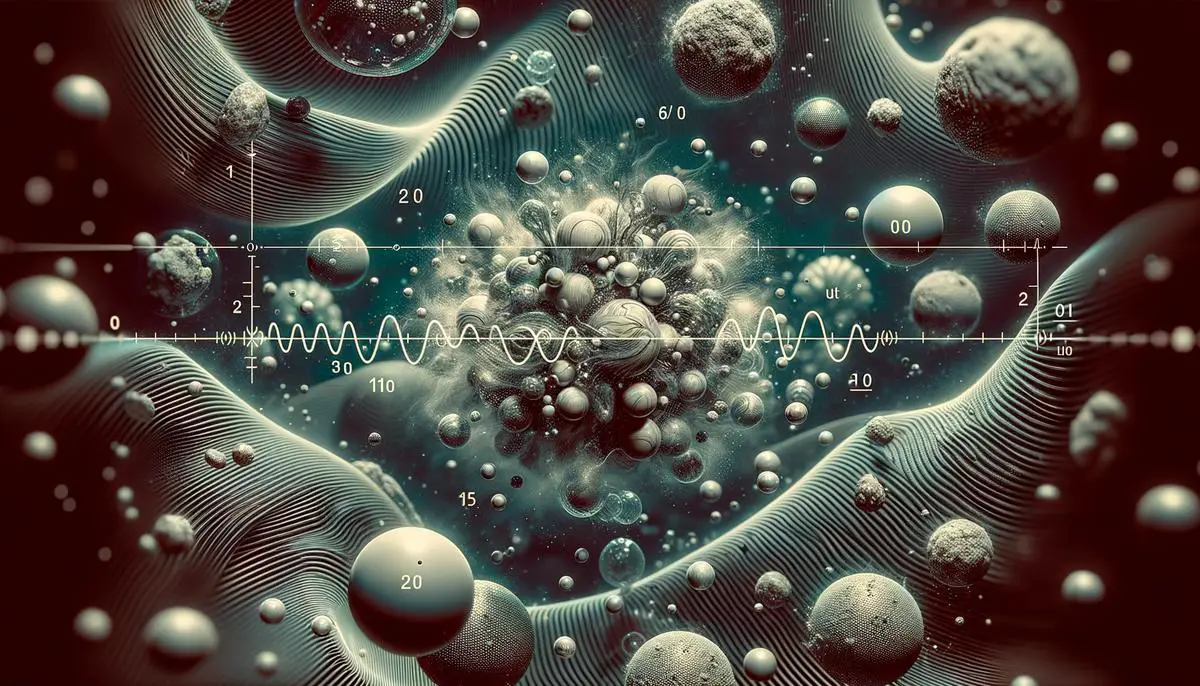
Forces at the Quantum Level
In the quantum realm, electromagnetism, governed by Coulomb's Law, plays a dominant role in atomic interactions, far outweighing the influence of gravity. This law describes how charged particles attract or repel each other, forming the basis for atomic stability.
However, the strong electromagnetic attraction between protons and electrons doesn't cause atoms to collapse due to the principles of quantum mechanics. The wave-particle duality allows electrons to exist in multiple states simultaneously, creating a probabilistic cloud around the nucleus rather than following a predictable path.
This quantum behavior ensures atomic stability, preventing electrons from falling into the nucleus despite the strong electromagnetic pull. The interplay between electromagnetic forces and quantum principles creates a delicate balance, allowing atoms to serve as the building blocks of matter while adhering to the unique rules of the quantum world.
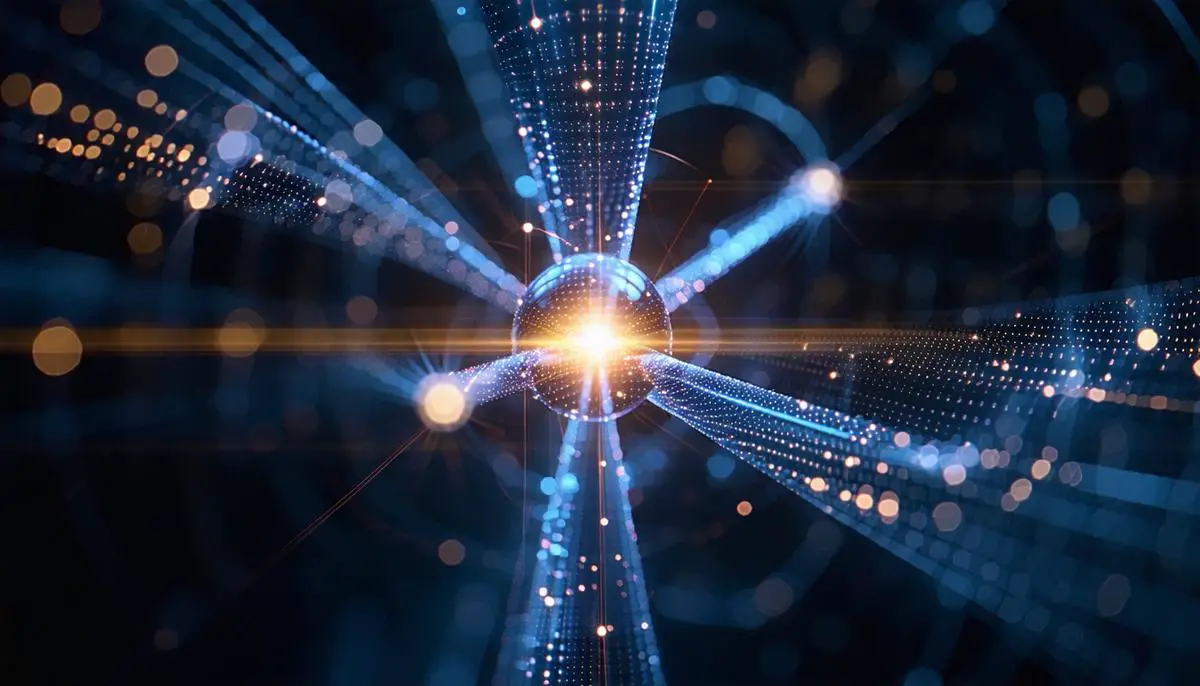
Quantum Phenomena Beyond the Atomic Scale
Quantum effects can manifest at larger scales through phenomena like superconductivity and superfluidity. These occurrences demonstrate how quantum principles can influence macroscopic behavior under specific conditions.
- Superconductivity: Electrons move through a material with zero electrical resistance. This coherent state allows for the frictionless flow of electricity, defying classical expectations.
- Superfluidity: Atoms organize into coordinated waves, enabling a liquid to flow without viscosity. This quantum alignment results in fluid behavior that transcends conventional fluid dynamics.
- Bose-Einstein condensates: At extremely low temperatures, certain particles form a single, massive quantum entity, exhibiting wave-like properties on a larger scale.
These macroscopic quantum phenomena highlight how the principles of quantum mechanics can extend beyond the atomic level, offering new perspectives on material behavior and opening avenues for technological applications.
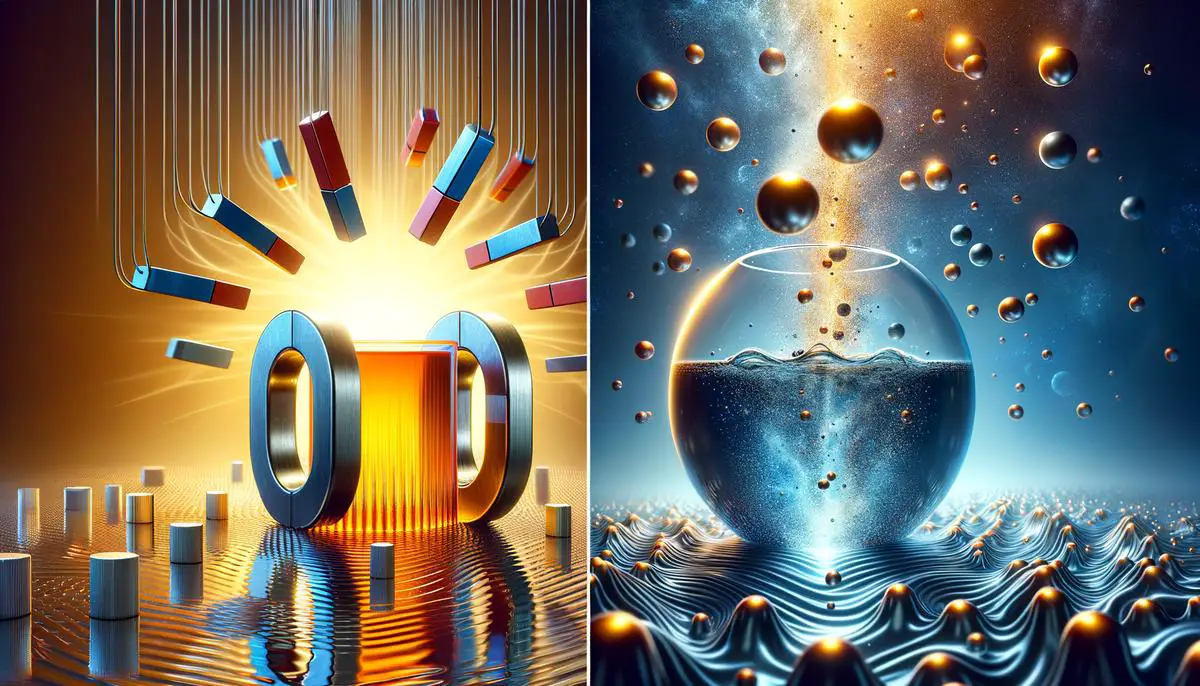
Quantum Entanglement in Protons
Recent discoveries have revealed that quantum entanglement exists within protons, challenging our previous understanding of these fundamental particles. This finding suggests that the quarks and gluons within protons are interconnected in ways that go beyond classical physics.
High-energy particle collisions have provided insights into this entanglement, showing that the constituents of protons influence each other through quantum relationships. This interconnectedness affects how we interpret the outcomes of particle collisions and our understanding of nuclear structure.
"Before we did this work, no one had looked at entanglement inside of a proton in experimental high-energy collision data," said Zhoudunming Tu, a Brookhaven Lab physicist.
The study of entanglement in protons has implications for nuclear physics and may lead to new insights into high-energy interactions. Upcoming facilities like Brookhaven Lab's Electron-Ion Collider will further explore how entanglement behaves in nuclear environments, potentially revealing new aspects of quantum behavior in matter.
This emerging field of study challenges existing models of particle physics and opens new avenues for understanding the fundamental nature of matter and the universe.
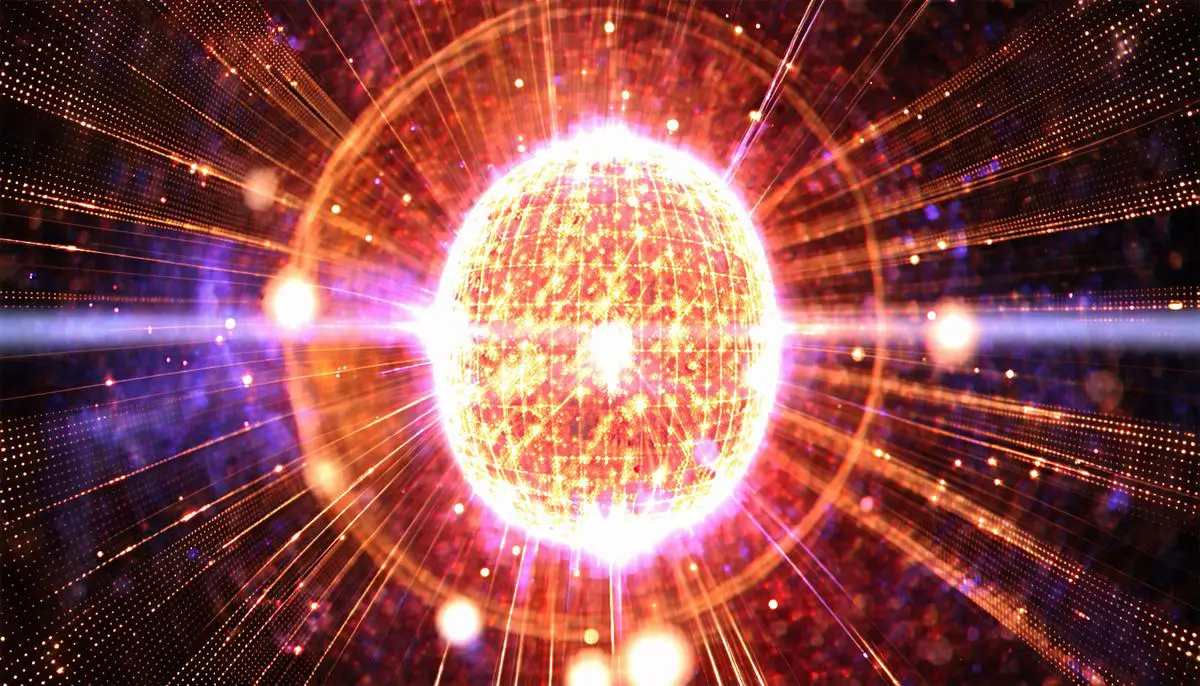
Advancements in Quantum Measurement Technologies
Recent developments in quantum measurement technologies are enhancing our ability to study and utilize quantum phenomena. Two notable advancements are SiCMag and chip-scale lasers.
SiCMag: A Cutting-Edge Magnetometer
- Uses silicon carbide to detect magnetic fields with high sensitivity
- Compact size and low power consumption
- Ideal for space exploration and planetary magnetic field studies
Chip-Scale Lasers
- Developed at UC Santa Barbara
- Significant miniaturization of laser technology
- High performance comparable to larger, more expensive systems
- Suitable for quantum computing and space-based quantum systems
These technologies have the potential to advance fields such as quantum computing, planetary science, and gravitational studies. They exemplify how innovations in quantum measurement are expanding our capabilities to explore and understand the universe at both quantum and cosmic scales.
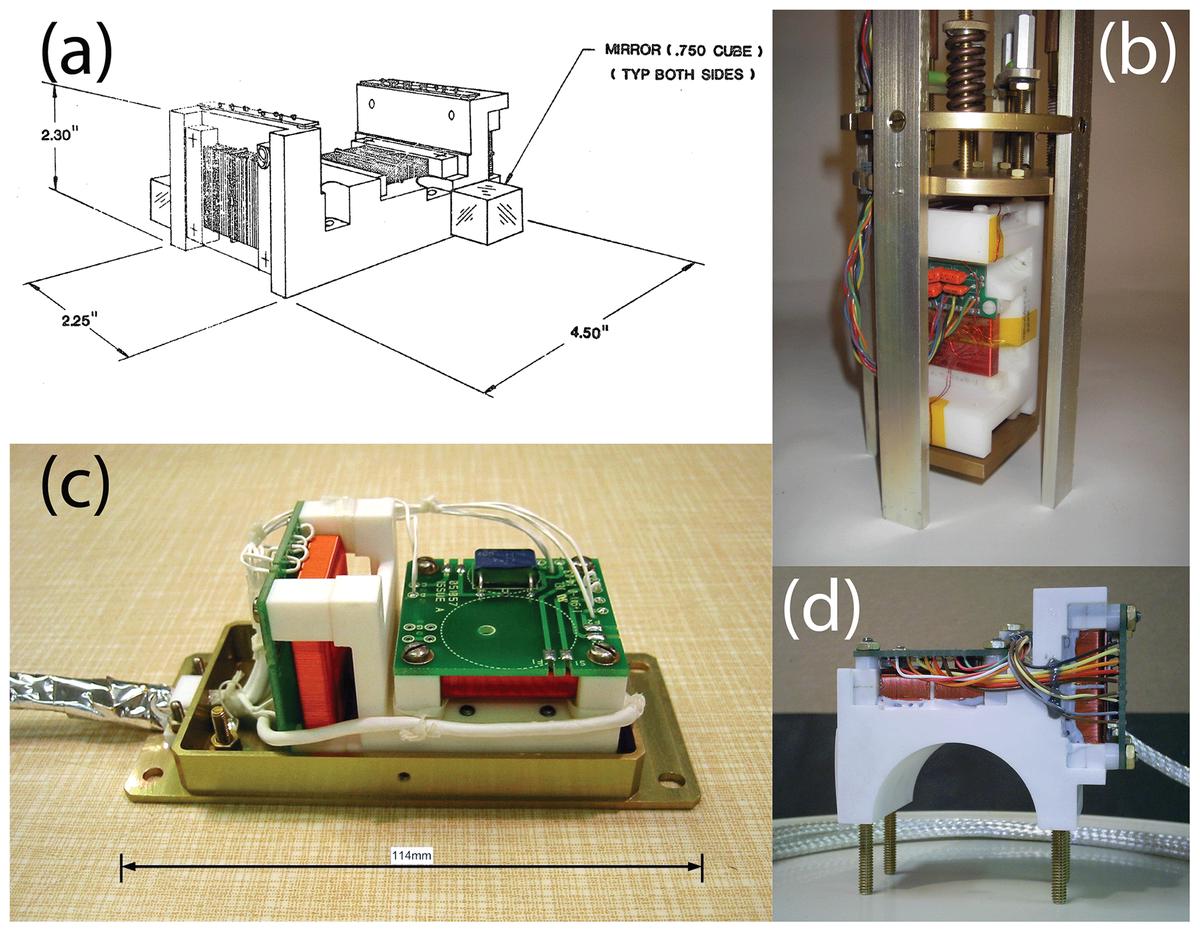
Quantum mechanics continues to reveal the intricate nature of the universe at its smallest scales, challenging our classical understanding of physics. As our measurement technologies advance, we gain deeper insights into quantum phenomena, opening new possibilities for scientific discovery and technological innovation.
- Isichenko A, Blumenthal D. Chip-scale ultra-low-linewidth self-injection locked 780 nm laser. Sci Rep. 2023.
- Tu Z, Kharzeev D, Schmitt S. Quantum entanglement inside protons. Rep Prog Phys. 2023.