Albert Einstein's contributions to physics have shaped scientific inquiry, especially his unexpected influence on quantum mechanics. His early work on the photoelectric effect and the dual nature of light set the stage for debates and discoveries that continue to challenge and inspire physicists today.
Einstein's Role in Quantum Mechanics
Albert Einstein inadvertently paved the way for quantum mechanics with his early contributions. His 1905 paper on the photoelectric effect proposed that light behaves not only as waves but also as particles—later known as photons. This radical idea explained why shining a dim light at a metal could cause electrons to jump off, earning Einstein a Nobel Prize.
Einstein explored the wave-particle duality, suggesting light was sometimes a wave and sometimes a particle. This concept laid the foundation for other scientists like Niels Bohr and established the beginning of quantum theory.
Later, Einstein found himself grappling with the emerging quantum ideas. He wasn't entirely convinced by randomness, which was becoming a key part of the new quantum mechanics. Einstein, who famously believed "God does not play dice," hoped for a more deterministic explanation.
His skepticism didn't stop him from making significant contributions. In 1916, he explained Max Planck's blackbody spectrum, connecting the interaction between photons and atoms. This was crucial because it helped further validate the particle nature of light and provided a stepping stone for the science that would eventually give us lasers and quantum technology.
Einstein and Bohr engaged in fierce debates over quantum concepts. While Bohr leaned into the unpredictability, Einstein held fast, looking for more concrete understandings. Their dialogue pushed the boundaries of physics, even if they often disagreed.
Later in his career, Einstein partnered with physicists Boris Podolsky and Nathan Rosen, which brought us the famous EPR paper. It presented the idea of entanglement, which says particles could be so interlinked that the actions of one could instantly affect the other—even if they were galaxies apart. Einstein saw this as a sign something was missing from quantum theory.
Despite his conflicts with the oddities of quantum mechanics, Einstein's legacy in the field remains monumental. His ideas and critiques fueled further explorations, leading to new insights and technologies that define physics today.
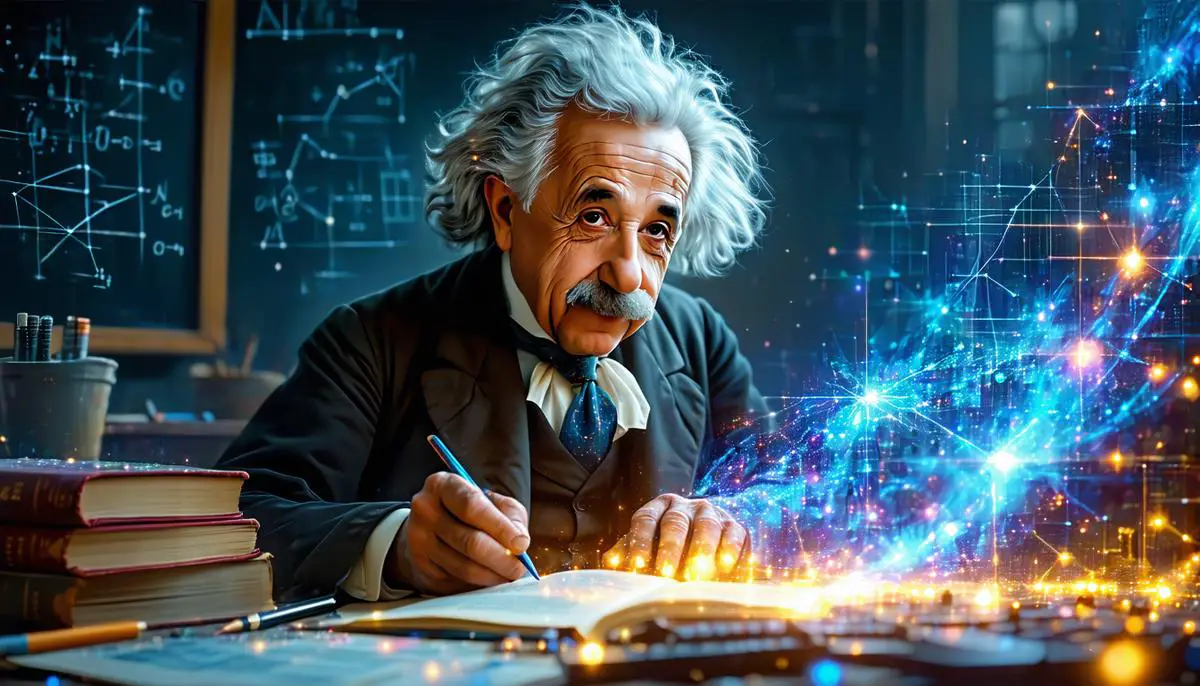
Einstein's Critique of Quantum Mechanics
Einstein's philosophical objections to the probabilistic nature of quantum mechanics were rooted in his quest for a deterministic universe. He persistently wrestled with the notion that quantum mechanics introduced a level of randomness that seemed contrary to the ordered and predictable universe he envisioned. In his debates with Niels Bohr, Einstein consistently argued against the intrinsic probabilism of quantum mechanics, suggesting that there must be more beneath the surface.
These discussions between Einstein and Bohr were philosophical battles over the nature of reality itself. While Bohr championed the idea that quantum mechanics described the limits of what could be known about physical phenomena, Einstein disagreed. He was unimpressed by a theory that did not allow for absolute predictability, asserting that a Theory of Everything should strive for completeness and determinism.
Central to Einstein's critique was his belief in hidden variables—unseen factors that he thought might account for the apparent randomness of quantum systems. He suspected that what seemed random was simply a reflection of our ignorance. The EPR paradox, a thought experiment prepared with Boris Podolsky and Nathan Rosen, was Einstein's way of highlighting what he saw as the inadequacies in quantum theory. Their work questioned whether quantum mechanics could be considered a complete theory of nature.
Einstein likened this perplexing "spooky action at a distance" to an incomplete map of reality, unable to explain how entangled particles remained connected without violating the speed limits set by relativity. Yet, instead of undermining the legitimacy of quantum mechanics, the EPR paradox laid the groundwork for future investigations into entanglement—a cornerstone concept in modern quantum physics.
In their exchanges, Bohr respectfully countered Einstein's concerns with the Copenhagen interpretation of quantum mechanics. He highlighted that quantum mechanics was coherent within its own framework, providing consistent predictions even if it seemed counterintuitive.
While Einstein never saw his preferred deterministic explanations materialize, his relentless probing of quantum mechanics paved the way for discoveries that form the foundation of technologies today.
Einstein's Legacy and Quantum Entanglement
Einstein's legacy in quantum mechanics is intricately tied to the concept of quantum entanglement, an idea that substantially emerged from his skeptical philosophy. Through his collaborations with Boris Podolsky and Nathan Rosen, Einstein's unease with quantum theory formalized in the EPR paper inadvertently became the catalyst for profound breakthroughs.
Instead of fading into obscurity with Einstein's criticisms, the concept of entanglement blossomed into a defining feature of quantum theory. It became a staple not just for its philosophical implications but for its experimental verifications, which pushed the boundaries of what was thought possible.
Einstein's apprehension about entanglement's 'spooky' nature did not deter experimenters from exploring it. Decades after the EPR paper, physicists like John Bell revisited the questions posed by Einstein, highlighting the truly non-classical nature of quantum mechanics. Bell's Theorem formulated testable inequalities that could discern whether local hidden variables could exist. Surprisingly, the experiments designed around Bell's Theorem, especially Alain Aspect's trials in the 1980s, reinforced the notion that entanglement was not just a theoretical quirk but an experimentally confirmable reality.
The implications of confirming entanglement were transformative, opening the doors to revolutionary technologies. In contemporary physics, the spooky action at a distance serves as a foundational principle in quantum computing and quantum cryptography. By exploiting the entangled states where particles remain interconnected irrespective of distance, these advancements promise unparalleled computational power and communication security.
Einstein's legacy is paradoxical in its essence—through querying what he saw as inadequacies, he unwittingly highlighted features of quantum mechanics that would drive its most astonishing developments. Even as he stood apart from his peers, his questions catalyzed a deeper understanding of the quantum world, proving that even skepticism, when directed with insight, can light the path of scientific discovery.
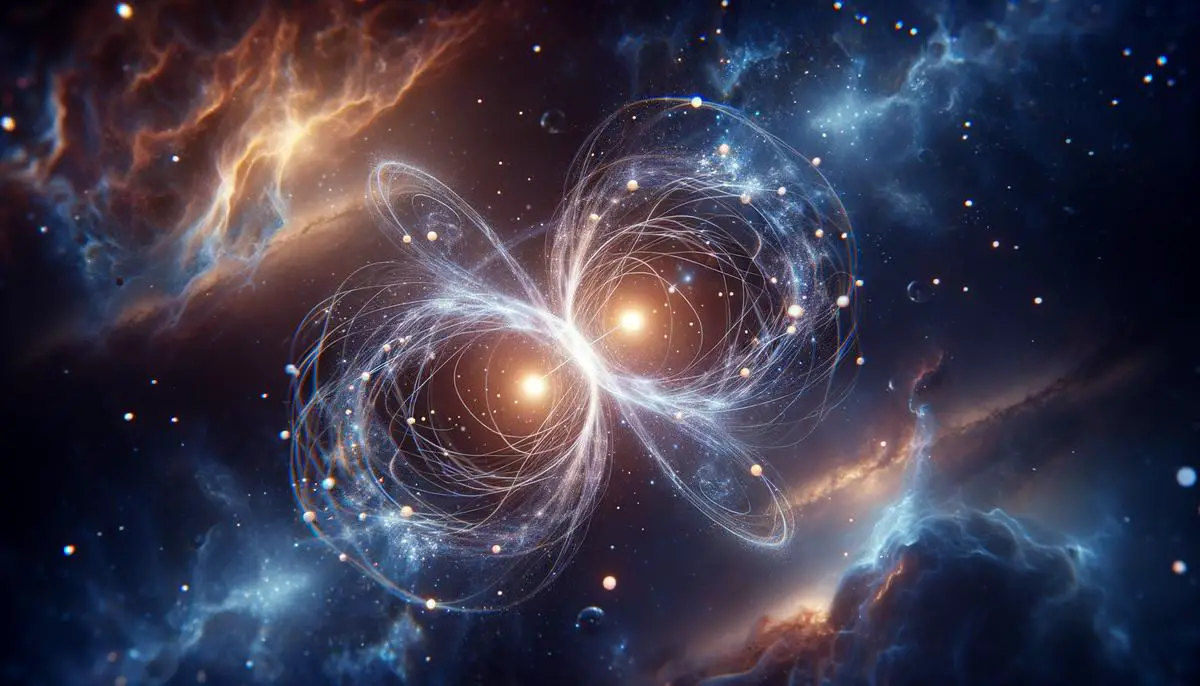
Modern Interpretations and Theories
Einstein's questions laid the groundwork for today's scientific endeavors that seek to marry the quantum world with the cosmic canvas of general relativity. As physicists work to reconcile these two paradigms, a new generation of theories and experiments strives to address the concerns Einstein voiced nearly a century ago.
One of today's exciting frontiers is quantum gravity, a field that attempts to merge quantum mechanics' probabilistic behavior with Einstein's deterministic framework of spacetime. The challenge has been significant; quantum physics dances with uncertainty while general relativity paints a picture of the universe as a precise, curvature-influenced structure.
Physicists at University College London are proposing theories that challenge old presumptions. A novel approach termed the "postquantum theory of classical gravity" suggests that spacetime can remain untouched by the bizarre quirks of quantum behavior, while intrinsic unpredictabilities, possibly rooted in spacetime's classical nature, influence quantum phenomena.
Researchers are exploring methods to observe potential disturbances in mass over time, a process that could validate these innovative theories. Such initiatives reflect a tribute to Einstein's curiosity and a commitment to sharpening the blurry edges of today's physics.
These postquantum perspectives extend their reach beyond gravity, hinting at new understandings regarding quantum measurement postulates. These developments propose that quantum superpositions naturally collapse through their interaction with classical spacetime, eliminating the need for conventional measurement-driven explanations.
Modern theoretical physicists probe these foundational questions and simultaneously venture towards resolving the enigmatic black hole information paradox—where quantum philosophy usually conflicts with relativistic theory. These explorations hint at spacetime's possible role in contextualizing what Einstein once saw as a conundrum too great to reconcile.
These pursuits promise concrete implications with the potential to revolutionize technology, mirroring the intent behind Einstein's original forays into quantum territory. Today's advancements continue to dance with Einstein's pivotal questions, pushing the boundaries of what we comprehend about how our universe fundamentally operates.
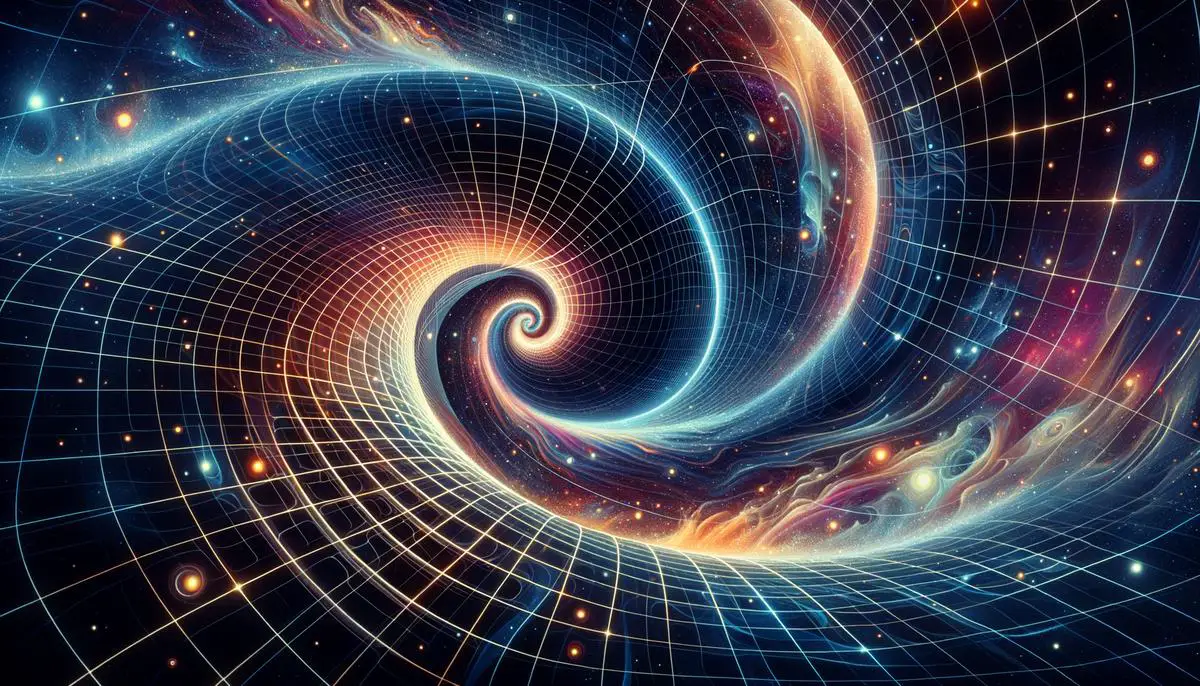
Einstein's legacy in quantum mechanics, marked by his probing questions and critical insights, continues to propel scientific exploration forward. His skepticism about quantum theory's randomness has sparked advancements that define contemporary physics, illustrating how even doubt can illuminate the path to understanding.
- Aspect A. Einstein and the Quantum Revolutions. Univ. Chicago Press; 2024.
- Fine A. The Shaky Game: Einstein, Realism and the Quantum Theory. University of Chicago Press; 1986.
- Howard D. Einstein on Locality and Separability. Studies in History and Philosophy of Science. 1985;16:171-201.
- List C, Pivato M. Emergent Chance. The Philosophical Review. 2015;124(1):119-152.