Understanding how astronomers discover exoplanets can be quite intriguing. Various methods, each with its own strengths and challenges, contribute to our growing knowledge of distant worlds. These techniques, from observing stellar wobbles to capturing direct images, offer unique insights into the vast diversity of planetary systems in our galaxy.
Radial Velocity Method
The radial velocity method, also known as the Doppler method, is a technique used by astronomers to find exoplanets. It detects the slight wobble of a star caused by the gravitational pull of an orbiting planet. This wobble is observed through changes in the star's light spectrum, with the light shifting towards blue when the star moves toward us and red when it moves away.
This method is particularly effective for detecting large planets close to their stars, as their gravitational pull is stronger. It has been especially successful in finding hot Jupiters, which are large gas giants orbiting very close to their stars.
The discovery of 51 Pegasi b in 1995 by Michel Mayor and Didier Queloz was a landmark achievement using this technique. This discovery, which earned them the 2019 Nobel Prize in Physics, revealed a new category of planets and opened up new avenues in exoplanet research.
Spectrometers are used to measure the tiny shifts in a star's light, helping astronomers determine the mass and orbit of the planet. To date, the radial velocity method has confirmed more than 5,500 exoplanets, each adding to our understanding of the diversity of planetary systems in our galaxy.
As technology advances, our ability to measure these subtle shifts continues to improve, paving the way for more discoveries and deeper insights into the nature of exoplanets.
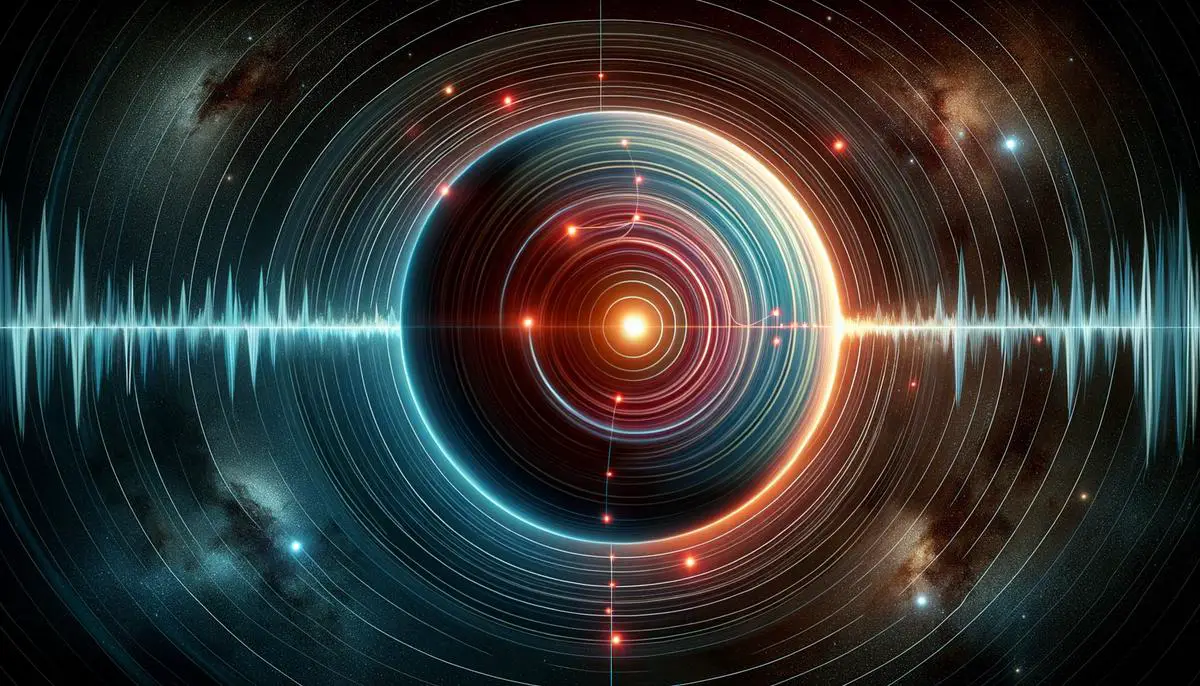
Transit Method
The transit method detects exoplanets by observing the slight dimming of a star's brightness as a planet passes in front of it. This technique has been particularly successful, with NASA's Kepler Space Telescope discovering more than 2,700 potential planets using this method.
A single transit can provide a wealth of information:
- The depth of the brightness dip indicates the planet's size
- The duration of the transit gives clues about its orbital period and distance from the star
The TRAPPIST-1 system, discovered using the transit method, exemplifies the power of this technique. It revealed seven Earth-sized planets orbiting a cool red dwarf star, with at least three in the habitable zone.
Transits can also provide information about a planet's atmosphere. As starlight passes through the planet's atmosphere during a transit, certain wavelengths are absorbed, creating a spectral fingerprint that can be analyzed to determine atmospheric composition.
The success of this method depends on the planet's orbit aligning with our line of sight and the host star exhibiting minimal intrinsic variability. While this limits the number of detectable transiting planets, the large number of stars observed increases the odds of detection.
Advanced telescopes like the James Webb Space Telescope are set to examine these spectral signatures in greater detail, potentially identifying biosignatures – chemical indicators of life.
Gravitational Microlensing
Gravitational microlensing utilizes Einstein's theory of general relativity to detect exoplanets. When a massive object passes in front of a distant star, its gravity acts as a lens, bending and magnifying the star's light. This temporary brightening of the star signals the presence of the intervening object, often a planet.
This method is particularly effective at detecting planets that are otherwise difficult to find, such as rogue planets – solitary worlds drifting through the galaxy unbound to any star. NASA's Nancy Grace Roman Space Telescope is expected to spot hundreds of these free-floating planets using microlensing.
Microlensing events are often transient and precise, lasting from hours to days and rarely repeating. This requires constant vigilance and quick, accurate measurements from astronomers.
"Gravitational microlensing allows us to detect planets thousands of light-years away within our Milky Way, as well as those in distant galaxies."
The first extragalactic planet candidate, M51-ULS-1b, was found in the Whirlpool Galaxy, 28 million light-years away, using this method.
Microlensing has helped uncover a variety of planetary compositions and orbits. For example, OGLE-2019-BLG-0960 b was discovered through this method, revealing a planet potentially 1.4 to 3.1 times the mass of Earth.
This approach demonstrates how fundamental principles of physics can be used to amplify faint signals from the cosmos, allowing us to detect planets that evade other methods.
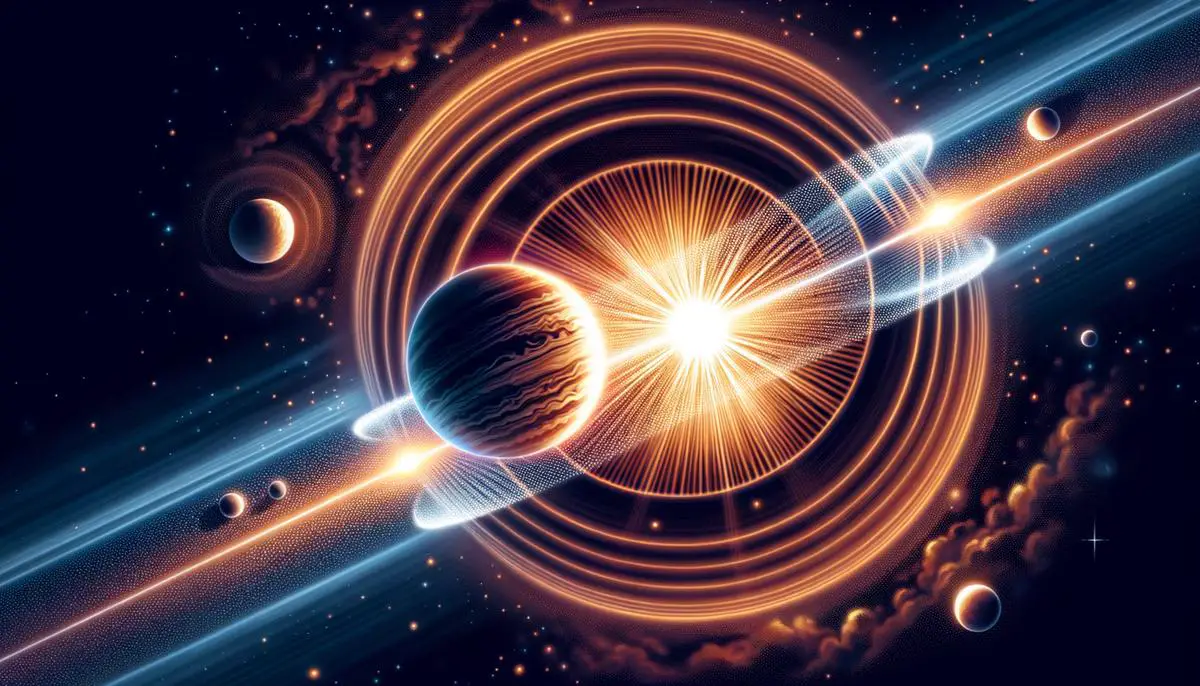
Direct Imaging
Direct imaging provides actual pictures of distant worlds orbiting other stars. This method faces significant challenges due to the extreme brightness difference between stars and their planets, often exceeding a factor of a million.
To overcome this, astronomers use coronagraphs, devices that block out the central light of a star, making the faint light from orbiting planets more discernible. This technique requires telescopes with exceptional resolution and sensitivity.
Ground-based observatories like the Very Large Telescope in Chile and the Keck Observatory in Hawaii, equipped with adaptive optics, have made significant strides in this field. The Hubble Space Telescope has also provided stunning exoplanet images from space.
Notable successes include:
- HR 8799 e: a gas giant several times the mass of Jupiter
- Beta Pictoris b: a young gas giant tracked over several years, providing insights into planetary formation and migration
- GJ 504 b: the "Pink Planet" that challenged existing models of planetary formation
Direct imaging is most effective for young, hot planets that emit considerable infrared radiation. Older, cooler planets are much harder to detect using this method.
Future advancements in telescope technology, such as the James Webb Space Telescope and the Extremely Large Telescope, are expected to enhance direct imaging capabilities. These next-generation telescopes will feature advanced coronagraphs and other cutting-edge instruments designed specifically for direct exoplanet imaging.
While direct imaging offers a unique perspective on exoplanets, it complements rather than replaces other detection methods. Each image provides a direct connection to these distant worlds, enriching our understanding of the universe's complexity and the diverse forms that planetary systems can take.
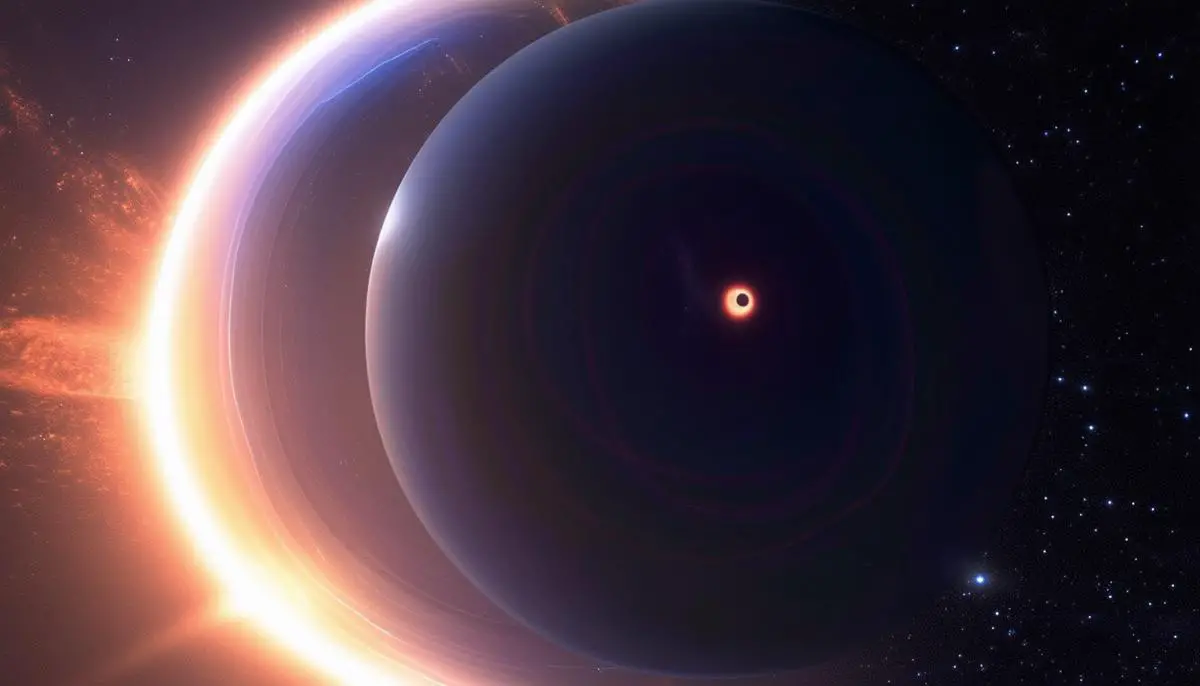
Astrometry
Astrometry is a method used to detect exoplanets by measuring a star's precise position in the sky. This technique observes the subtle wobble of a star caused by the gravitational pull of orbiting planets. The challenge lies in detecting extremely small movements, comparable to observing a hair's breadth movement from miles away.
While centuries old, astrometry has only recently become viable due to technological advancements. The need for extreme precision made it largely theoretical until the advent of space-based observatories. ESA's Gaia mission, launched in 2013, is at the forefront of this resurgence. Gaia's ability to track stellar positions with microarcsecond accuracy enables it to identify the subtle wobbles indicative of unseen planetary companions.
Astrometry's potential for future discoveries is significant, especially as Gaia continues to deliver high-precision data. It offers unique advantages:
- Detection of very long-period exoplanets (decades or centuries to orbit)
- Enrichment of our understanding of planetary system architectures
- Insights into star-planet interactions over lengthy periods
Although astrometry currently accounts for only a handful of exoplanet detections, its contribution to our understanding of the galaxy is invaluable. Future missions aim to further refine this technique, potentially revealing planetary systems around stars many light-years away and providing a clearer picture of the variety and complexity of planets in the universe.
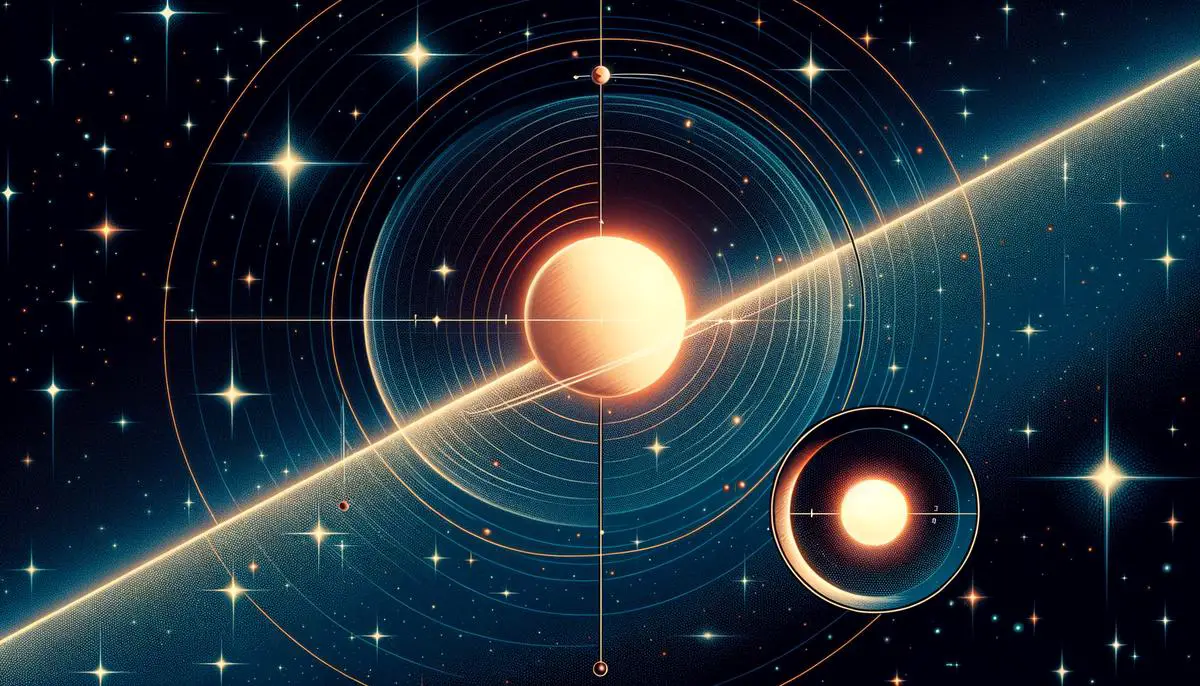
Emerging Techniques and Future Prospects
The future of exoplanet discovery is unfolding with innovative techniques and advanced missions. One promising approach involves using radio observatories like the Atacama Large Millimeter/submillimeter Array (ALMA) to detect gaps in debris disks around young stars, indicating the presence of forming planets.
The upcoming Nancy Grace Roman Space Telescope, scheduled for launch in the mid-2020s, promises to revolutionize exoplanet searches. It will employ various methods, including microlensing, to discover thousands of new exoplanets, particularly rogue planets and those in crowded star fields.
Next-Generation Telescopes and Instruments
- James Webb Space Telescope (JWST): Designed to study exoplanet atmospheres in unprecedented detail
- CARMENES instrument: Pivotal in hunting for terrestrial-type exoplanets around nearby red dwarf stars
Advancements in artificial intelligence and machine learning are accelerating exoplanet detection and analysis. These technologies help sift through vast datasets, identifying potential exoplanetary signals with greater efficiency and accuracy, and can predict which star systems are most likely to host planets.
"As we refine our techniques and develop new technologies, we are not only discovering new worlds but also beginning to understand their complex environments."
From nascent planets in debris disks to detailed atmospheric compositions of distant worlds, each discovery adds a profound piece to the cosmic puzzle, bringing us closer to answering fundamental questions about life in the universe.1
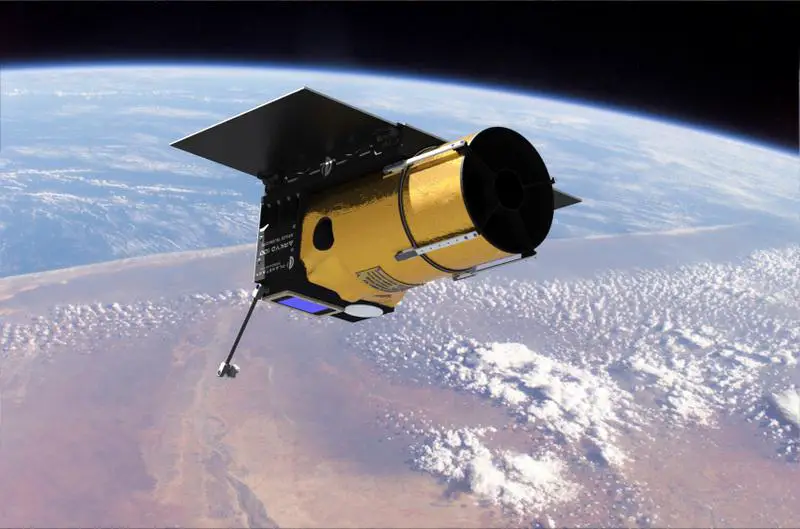
The quest to find and understand exoplanets continues to expand our knowledge. Each method plays a crucial role in revealing the universe's hidden wonders. As technology advances, our ability to uncover and study these distant worlds will only grow, bringing us closer to answering profound questions about our place in the cosmos.