Dark matter, an invisible force that makes up over 80% of the universe's total mass, has intrigued scientists for decades. Its gravitational effects on visible matter hint at its presence, yet it remains one of the most elusive components of our cosmos. From the pioneering observations of Fritz Zwicky in the 1930s to today's advanced space telescopes and particle colliders, the quest to understand dark matter continues to push the boundaries of astrophysics and cosmology.
Importance of Dark Matter
Dark matter constitutes more than 80% of the universe's total mass, despite neither emitting nor reflecting light. Scientists infer its existence from its gravitational pull on visible matter. This invisible substance is crucial in holding galaxies together, preventing them from disintegrating.
Swiss astronomer Fritz Zwicky first proposed the concept of dark matter in the 1930s while observing the Coma Cluster. He noted that the cluster's visible mass alone couldn't account for the gravitational forces keeping it intact. Today, dark matter remains one of the most enigmatic puzzles in astrophysics.
The behavior of galaxies defies the gravitational pull that visible mass can exert. Stars at the edges of galaxies rotate as fast as those near the center, suggesting an unseen force at play. Without dark matter acting as cosmic "glue," galaxies wouldn't exist in their current form.
Dark Matter Detection Projects
- NASA's Fermi Gamma-ray Space Telescope
- Large Hadron Collider
- Hubble Space Telescope
- Euclid Space Telescope
- Nancy Grace Roman Space Telescope
- James Webb Space Telescope
- Large Underground Xenon dark-matter experiment (LUX)
- IceCube Neutrino Observatory
Despite never having detected it directly, dark matter's gravitational effects on normal matter are indisputable. The search involves several ambitious projects. NASA's Fermi Gamma-ray Space Telescope scans the cosmos for gamma rays that might be produced when dark matter particles annihilate each other.
The Large Hadron Collider plays a significant role in this puzzle. Scientists speculate that colliding protons at high energies might produce transient dark matter particles, potentially helping to understand the particle physics underlying this elusive substance.
NASA has equipped missions like the Hubble Space Telescope to study dark matter indirectly through gravitational lensing. Hubble's observations of the Bullet Cluster provided crucial evidence: two colliding galaxy clusters showed a separation between visible mass and dark matter, reinforcing the latter's existence.
Newer missions amplify our hunt for dark matter. The Euclid Space Telescope aims to map dark matter across the universe by tracking the distortion of light traveling from distant galaxies. The Nancy Grace Roman Space Telescope will study dark energy, dark matter's cosmic counterpart, potentially revolutionizing astrophysics.
The James Webb Space Telescope, while primarily focused on viewing the universe in infrared, might reveal hidden clues about dark matter by observing the earliest light from small galaxies.
NASA collaborates with various international projects, including underground experiments like the Large Underground Xenon dark-matter experiment (LUX) and the IceCube Neutrino Observatory. These varied approaches underscore the multidisciplinary effort required to unravel dark matter's mysteries.
Dark matter's gravitational influence shapes the large-scale structure of the universe, governing the formation and evolution of galaxies and galaxy clusters. NASA's dedication to studying dark matter reflects its importance in advancing our understanding of the cosmos.
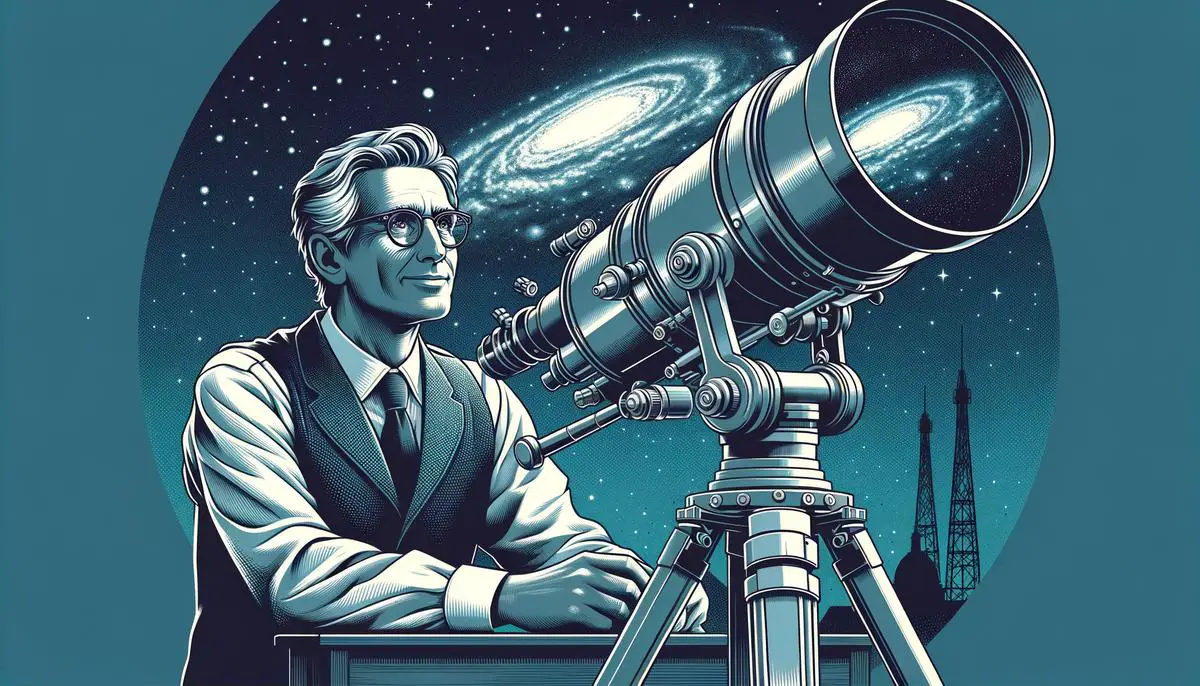
Methods of Detecting Dark Matter
Scientists employ various methods to detect dark matter, each utilizing sophisticated technologies and methodologies:
- Direct detection: Aims to capture rare instances when dark matter particles might collide with atomic nuclei in detectors. The Large Underground Xenon (LUX) experiment uses a highly purified liquid xenon target housed deep underground to shield it from ambient cosmic radiation. Any potential interactions between dark matter particles and xenon atoms could produce light and electrons detectable by sensitive sensors.
- Indirect detection: Looks for secondary evidence of dark matter interactions, such as cosmic phenomena resulting from dark matter particle annihilation or decay. NASA's Fermi Gamma-ray Space Telescope exemplifies this method, scanning for gamma rays that could emerge from these elusive interactions.
- Particle colliders: The Large Hadron Collider (LHC) in Switzerland attempts to create conditions similar to the early universe by colliding protons at extremely high energies. Scientists analyze the missing energy and momentum after collisions for signs that dark matter particles have been generated.
Space-based telescopes play a significant role in dark matter research. NASA's Hubble Space Telescope provides invaluable gravitational lensing data, allowing researchers to map dark matter distribution with precision. The Euclid Space Telescope focuses on mapping dark matter throughout the universe by observing light distortion from distant galaxies.
Laboratory experiments like XENON1T strive to observe dark matter particles directly by detecting potential interactions underground. The IceCube Neutrino Observatory in Antarctica tracks neutrinos, which might be linked to dark matter.
Particle physics research includes theoretical frameworks and computational models to predict dark matter behavior under different conditions, guiding experimental designs and observations.
"These varied detection methods exemplify a multi-pronged approach to understanding dark matter, showcasing the breadth and depth of scientific endeavor. Though definitive detection remains elusive, each experiment refines our understanding of the universe and drives technological advancements in the field."
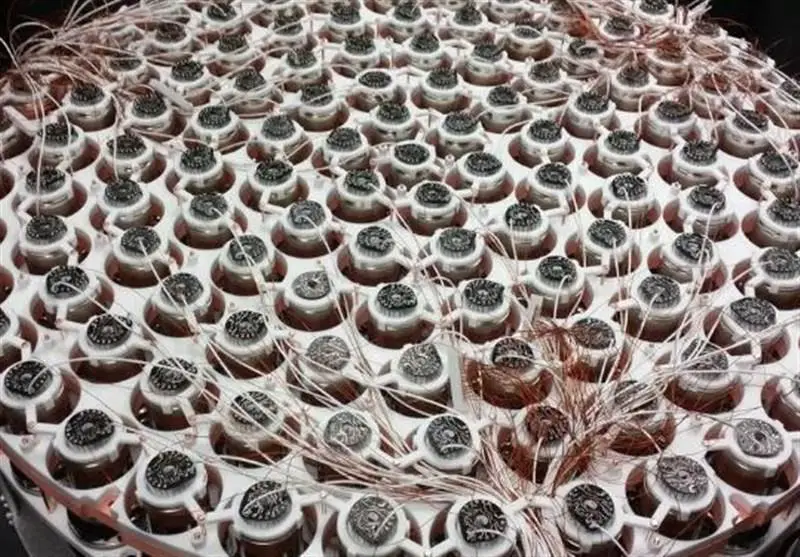
NASA's Key Missions and Instruments
NASA employs several key missions and instruments in its investigation of dark matter:
Mission | Key Features | Contribution to Dark Matter Research |
---|---|---|
Hubble Space Telescope (HST) | Advanced Camera for Surveys, Wide Field Camera 3 | Gravitational lensing data, mapping dark matter distribution |
James Webb Space Telescope (JWST) | High-resolution infrared imaging | Examining faintest and most ancient light sources |
Euclid Space Telescope | Visible-light imager (VIS), near-infrared spectrometer and photometer (NISP) | Tracing geometry of dark universe, creating extensive maps |
Nancy Grace Roman Space Telescope | Large field of view | Generating expansive, high-resolution maps of dark matter |
The Hubble Space Telescope (HST) has significantly contributed to our understanding of dark matter through its Advanced Camera for Surveys and Wide Field Camera 3. Hubble has provided detailed gravitational lensing data by capturing images of galaxy clusters like Abell 1689 and the Bullet Cluster, mapping dark matter distribution with high accuracy1.
The James Webb Space Telescope (JWST), launched in December 2021, expands on Hubble's legacy with its high-resolution infrared imaging capabilities. By examining the faintest and most ancient light sources, JWST helps researchers refine theoretical models of dark matter and its interactions with visible matter2.
The Euclid Space Telescope, launched in July 2023, is specifically designed to trace the geometry of the dark universe. Using its visible-light imager (VIS) and near-infrared spectrometer and photometer (NISP), Euclid creates extensive maps showing the distribution of galaxies and their dark matter halos over time. These maps help scientists understand the formation and evolution of cosmic structures3.
The Nancy Grace Roman Space Telescope, anticipated to launch by May 2027, will build on the foundations laid by Hubble and Euclid but with a significantly larger field of view. Roman will generate expansive, high-resolution maps of dark matter in the universe, providing detailed observations of gravitational lensing and offering new insights into the nature of dark energy4.
NASA's multi-mission approach underscores its commitment to solving the dark matter enigma. Through the combined capabilities of these telescopes, NASA is spearheading efforts to map the dark cosmos and understand its mysterious components. Each mission leverages unique technologies and observation strategies to fill crucial gaps in our knowledge, pushing the boundaries of astrophysics and cosmology.
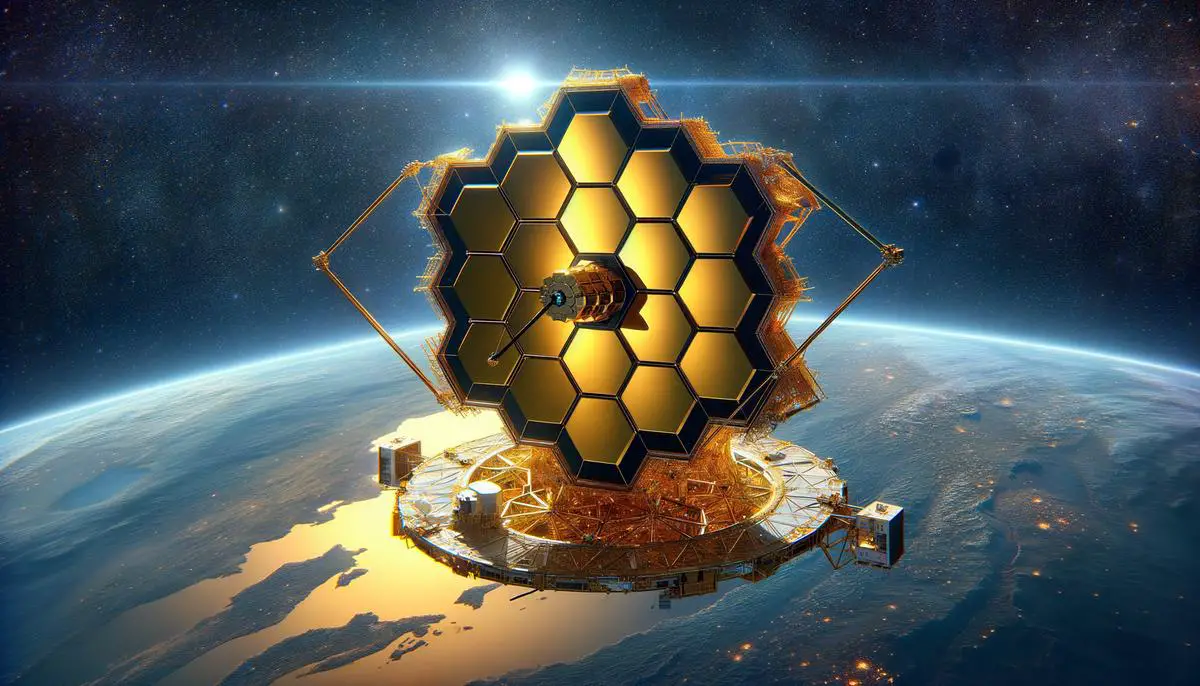
Challenges and Technological Innovations
Detecting and studying dark matter presents significant hurdles, primarily due to its elusive nature. Dark matter doesn't interact with electromagnetic forces, making it invisible to traditional sensors and detectors. This invisibility requires researchers to rely on indirect methods, such as observing its gravitational effects on visible matter, which demands highly sensitive and specialized instruments.
Ultra-sensitive detectors have been developed to capture rare interactions between dark matter particles and regular matter. These detectors, often located deep underground to shield them from cosmic radiation, are designed to pick up the faintest signals. Advances in cryogenics and materials science have been crucial in building these detectors. For instance, the Large Underground Xenon (LUX) experiment uses highly purified liquid xenon to detect possible interactions with dark matter particles.
The Large Hadron Collider (LHC) aims to recreate conditions similar to those just after the Big Bang by smashing protons together at unprecedented energies. This process could produce dark matter particles as byproducts of these high-energy collisions. Analyzing these events requires cutting-edge computational algorithms to sift through vast amounts of data, looking for anomalies that may indicate the presence of dark matter.
Space telescopes like NASA's Hubble and the James Webb Space Telescope (JWST) utilize gravitational lensing to observe the subtle distortions caused by dark matter clumps. Advances in imaging technology, such as improved sensors and adaptive optics, have made it possible to capture incredibly detailed and accurate images of these phenomena. This level of precision allows scientists to map dark matter more accurately than ever before.
The techniques developed for studying dark matter have found applications in various fields:
- Quantum technologies
- Medical imaging
- Atomic clock precision
- Gravitational wave detection
For example, highly sensitive detectors used in dark matter experiments have been adapted to enhance the precision of atomic clocks and improve the sensitivity of gravitational wave detectors. In medical imaging, the principles of particle detection and signal processing are being repurposed to improve PET (positron emission tomography) scans, making them more accurate and reducing the required radiation exposure.
These technological advancements demonstrate the interconnected nature of scientific discovery, where progress in one area can catalyze breakthroughs in others. As the quest to understand dark matter continues, the tools and technologies developed will undoubtedly find new and unforeseen uses, highlighting the far-reaching impact of astrophysical research.
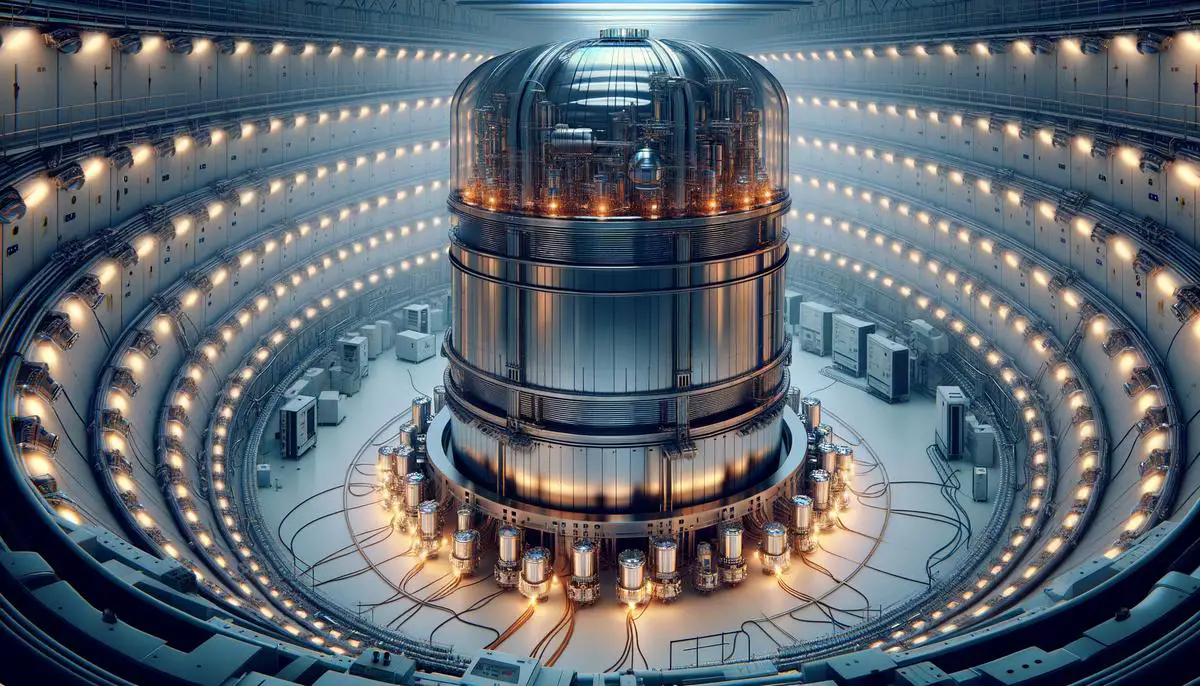
Future Prospects and Collaborations
The future of dark matter research is promising, with several ambitious missions and collaborations on the horizon. NASA, alongside international partners, is preparing to launch innovative projects that may unravel the secrets of this mysterious entity.
Key upcoming missions include:
- Nancy Grace Roman Space Telescope: Scheduled for launch by May 2027, it will take observational cosmology to new heights with its wider field of view and higher sensitivity.
- Vera C. Rubin Observatory: Expected to be operational by 2025, it will conduct the Legacy Survey of Space and Time (LSST) in Chile.
- Euclid mission: A joint venture between NASA and the European Space Agency, exemplifying international collaboration.
The upgraded Large Hadron Collider (LHC) continues its quest to detect dark matter by simulating conditions akin to the universe's infancy. Future upgrades aim to increase the collider's luminosity, improving the chances of observing fleeting dark matter particles. Simultaneously, subterranean experiments like XENON1T and its successors will search for direct interactions with dark matter particles in isolated environments.
For aspiring scientists interested in contributing to this field, a strong foundation in several areas is crucial:
- Physics
- Mathematics
- Engineering
- Programming
- Data analysis
- Computational modeling
Interdisciplinary knowledge can also prove valuable, as dark matter research often intersects with other domains such as quantum mechanics, astrophysics, and materials science.
Engaging with the scientific community through conferences, workshops, and collaborations is important for networking and academic growth. Institutions worldwide offer specialized programs and workshops designed to train the next generation of astrophysicists and cosmologists.
"As missions like Roman and Rubin prepare to expand our understanding, international collaborations will amplify the reach and impact of their discoveries. The scientific community eagerly anticipates the breakthroughs that these future initiatives will bring, pushing the boundaries of our cosmic knowledge ever further."
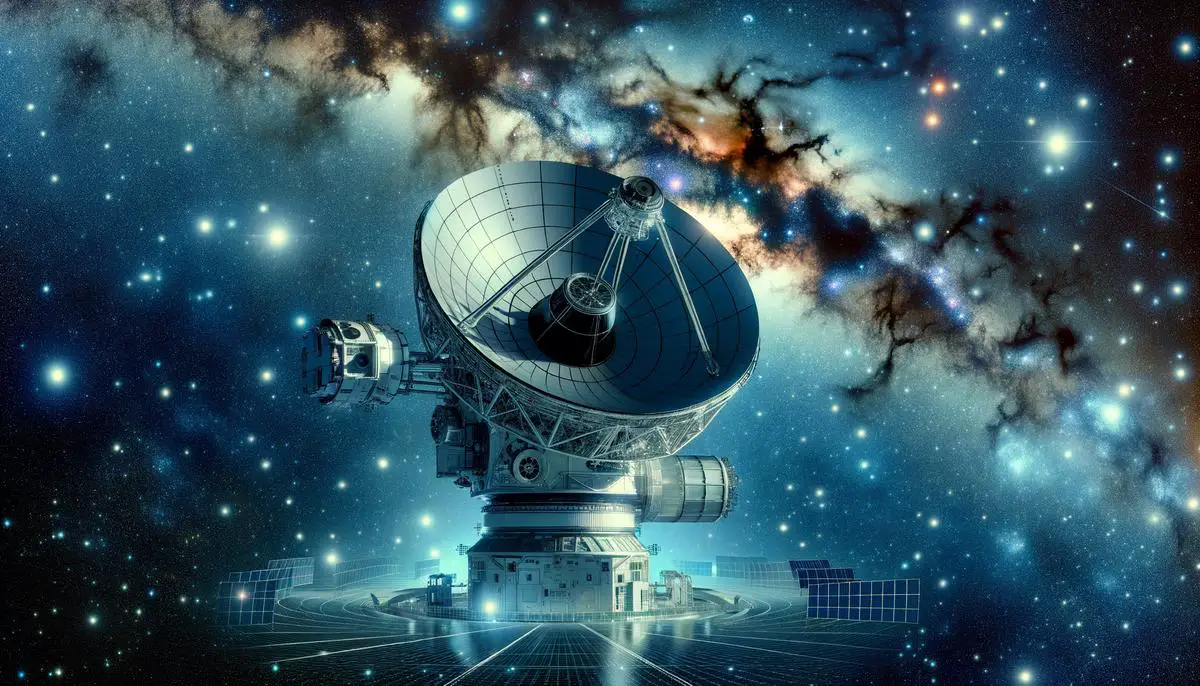
Dark matter's gravitational influence shapes the large-scale structure of the universe, governing the formation and evolution of galaxies and galaxy clusters. NASA's dedication to studying dark matter underscores its importance in our understanding of the cosmos. This invisible force continues to drive the field of astrophysics forward, promising profound insights into the nature of our universe.