Black holes, with their immense gravitational pull and mysterious nature, have long intrigued scientists and the public alike. Understanding how these cosmic phenomena form and influence their surroundings offers insights into some of the most extreme conditions in the universe.
Formation of Black Holes
Black holes are born through two primary processes: stellar collapse and direct gas collapse. These mechanisms are rooted in the laws of physics and the universe's natural behavior.
Stellar Collapse
When a massive star exhausts its nuclear fuel, it can no longer sustain its outward pressure against gravity, leading to a violent collapse. For stars with a mass at least three times that of our Sun, this collapse compresses the core to a point of zero volume and infinite density, forming a singularity.
The star's outer layers are often blown away in a supernova explosion, which precedes the black hole's birth. The singularity at the center, hidden by the event horizon, exerts such strong gravitational pull that even light cannot escape. The radius of this event horizon depends on the mass of the collapsed star, often termed as the Schwarzschild radius.
Stars with lower masses evolve differently, ending their lives as white dwarfs or neutron stars.
Direct Gas Collapse
In the early universe, supermassive black holes could form through direct gas collapse. This process involves colossal clouds of gas collapsing directly under their gravity, bypassing the star formation stage.
Under the right conditions, a cloud with a mass equivalent to millions of Suns can collapse rapidly. The gravitational forces compress the gas to incredibly high densities, leading to the formation of a supermassive black hole within about 150 million years.
This method of formation explains how some quasars, observed more than 12 billion years ago, already possessed supermassive black holes.
Stellar Black Holes vs. Supermassive Black Holes
While both types share the fundamental trait of an event horizon, their growth pathways differ significantly. Stellar black holes are relatively small, often a few times the mass of the Sun, and grow by accreting nearby matter. Supermassive black holes, found at the centers of galaxies, result from either direct gas collapse or the merging of smaller black holes over time.
Mergers and Growth
Black holes can merge when orbiting each other closely. They emit gravitational waves, losing energy until they collide and form a single, larger black hole. This process, confirmed by the Laser Interferometer Gravitational-Wave Observatory (LIGO) since 2015, demonstrates the dynamic nature of the universe and the intricate interplay of gravity on a cosmic scale.1
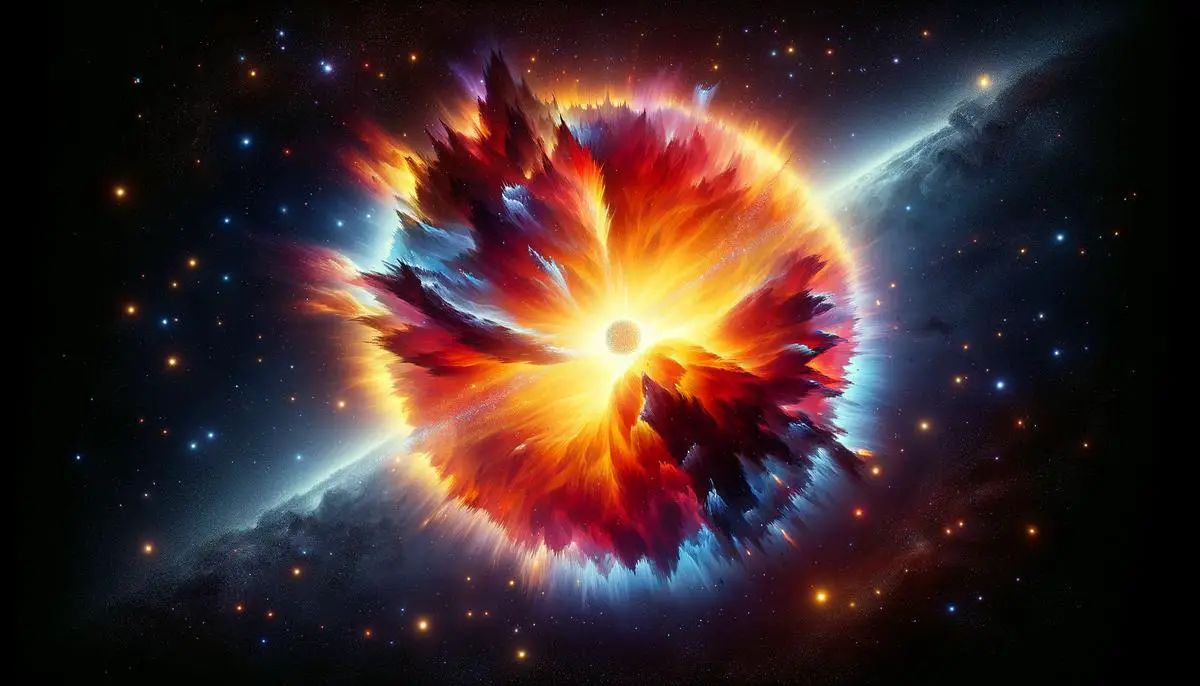
Types of Black Holes
Black holes come in various sizes, each with distinct characteristics and formation scenarios:
- Stellar black holes emerge from the remnants of massive stars. They typically possess a mass ranging from a few to several times that of our Sun. Despite their relatively small size, stellar black holes exhibit exceptionally high density and strong gravitational pull, accreting nearby gas and dust to grow incrementally.
- Supermassive black holes lurk at the heart of virtually every large galaxy, including our Milky Way. These giants range from millions to billions of solar masses. Their formation remains an intriguing mystery, with theories including the merger of numerous smaller black holes or the direct collapse of massive gas clouds. Supermassive black holes exert a profound influence on their host galaxies, often powering quasars and active galactic nuclei.
- Intermediate black holes occupy the middle ground between stellar and supermassive black holes, with masses ranging from hundreds to hundreds of thousands of times that of the Sun. These elusive entities may form through the collision and merger of stars in dense clusters or represent a stepping stone in the growth of supermassive black holes. Evidence for their existence has been mounting, though they remain less well-understood than their smaller and larger counterparts.
Each category of black hole contributes to our understanding of cosmic evolution and the extremes of astrophysics.
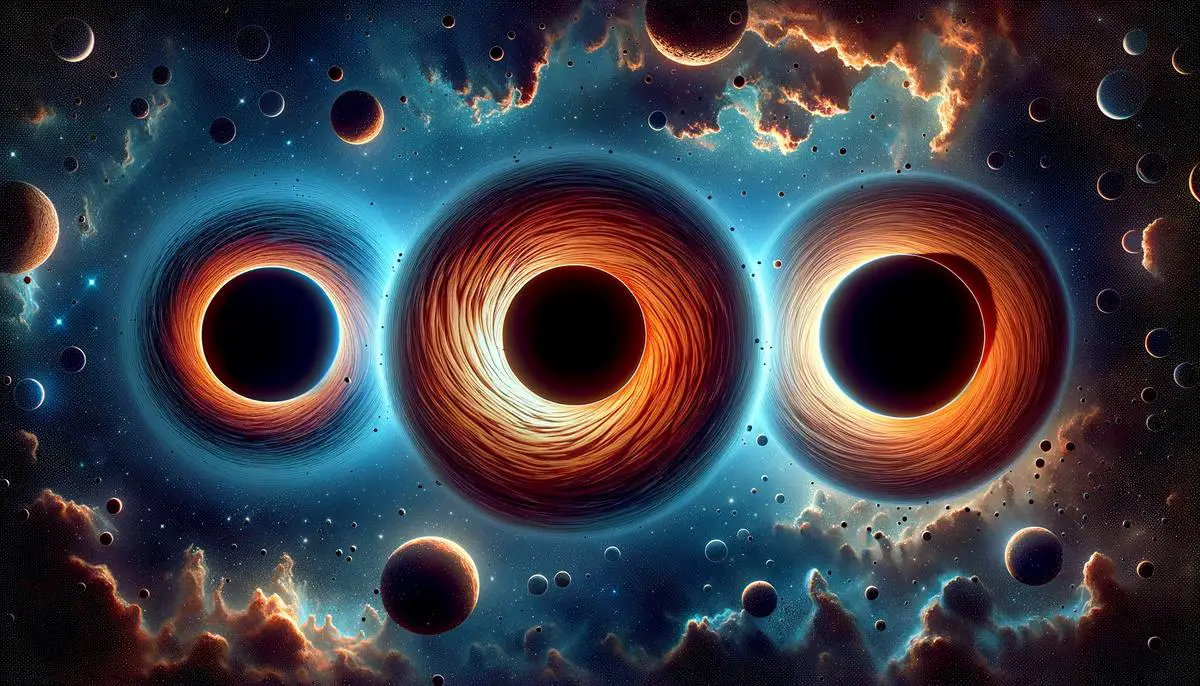
Detection and Observation
Despite their invisible nature, scientists have developed methods to infer the presence of black holes and study their properties through indirect means:
- Gravitational effects: By observing the orbits of visible objects, astronomers can detect the presence of an unseen massive object. In binary star systems, a black hole can be inferred by its gravitational influence on its visible companion.
- X-ray emissions: When a black hole accretes material from a companion star, the infalling matter forms a hot accretion disk that emits X-rays. Space-based observatories like NASA's Chandra X-ray Observatory study these emissions.
- Advanced telescopes: The Event Horizon Telescope (EHT) project captured the first-ever direct image of a black hole's event horizon in 2019, visualizing the "shadow" of the supermassive black hole in the M87 galaxy.2
- Gamma-ray detection: Instruments like the Very Energetic Radiation Imaging Telescope Array System (VERITAS) detect high-energy gamma rays, providing clues about extreme astrophysical events often involving black holes.
- Gravitational waves: The Laser Interferometer Gravitational-Wave Observatory (LIGO) detects ripples in space-time caused by merging black holes, confirming the existence of stellar-mass black holes in binary systems.
- Star dynamics: Observations of stars orbiting galactic centers, such as those around Sagittarius A* in the Milky Way, provide evidence for supermassive black holes.
These methods combine to provide a comprehensive understanding of black holes, enriching our knowledge of the universe's most extreme phenomena.
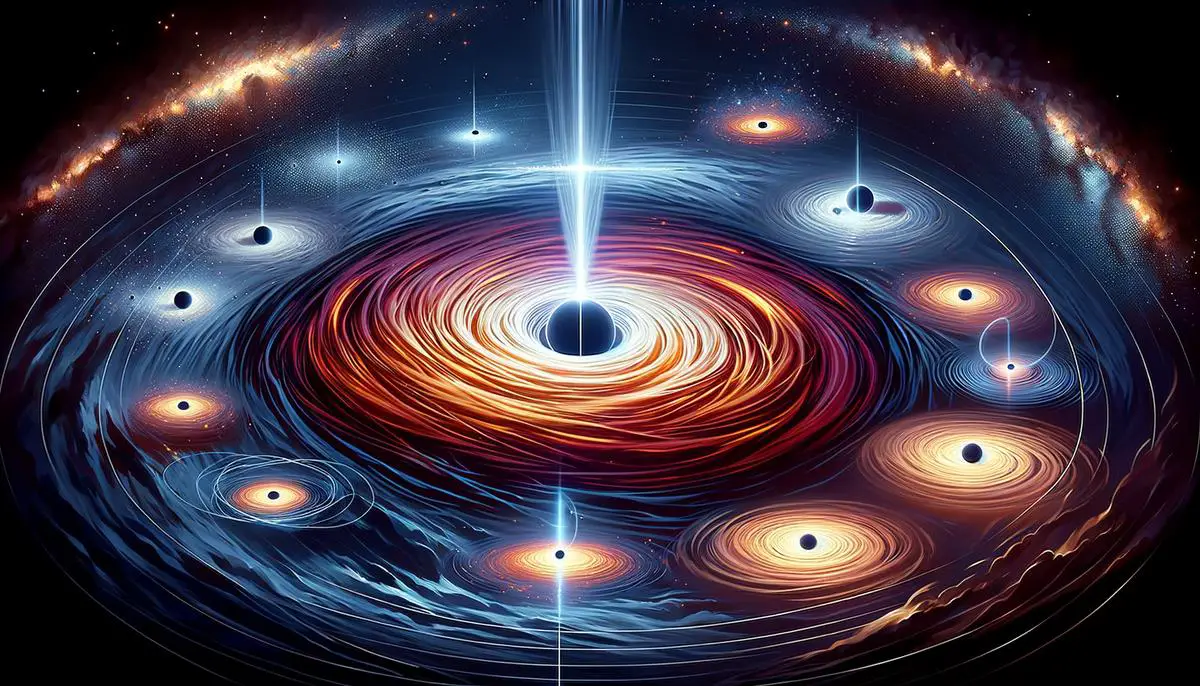
Effects on Surroundings
Black holes exert a profound influence on their cosmic environment:
- Accretion disks: When matter falls towards a black hole, it often forms a swirling accretion disk. As material spirals inward, it heats up due to friction and gravitational compression, emitting electromagnetic radiation, particularly X-rays.
- Relativistic jets: Magnetic fields within the accretion disk can funnel some material into narrow jets that shoot out perpendicular to the disk at nearly light speed. These jets transport energy and matter across vast cosmic distances, influencing the interstellar medium.
- Galactic influence: Supermassive black holes at galactic centers can regulate star formation through a process called "feedback." Their energy output can heat surrounding gas, preventing it from cooling and forming new stars. Conversely, under certain conditions, black hole outflows can compress gas and trigger star formation.
- Space-time warping: The intense gravity of black holes distorts space-time, affecting the orbits of nearby stars and providing clues about the black hole's mass and influence.
- Cosmic reionization: During the early universe, the intense radiation from quasars (powered by supermassive black holes) contributed to the reionization of the cosmos, playing a vital role in its evolution.3
From shaping galactic evolution to influencing the state of the early universe, black holes are key players in cosmic processes. Their effects illustrate how extreme physics can have far-reaching consequences across astronomical distances.
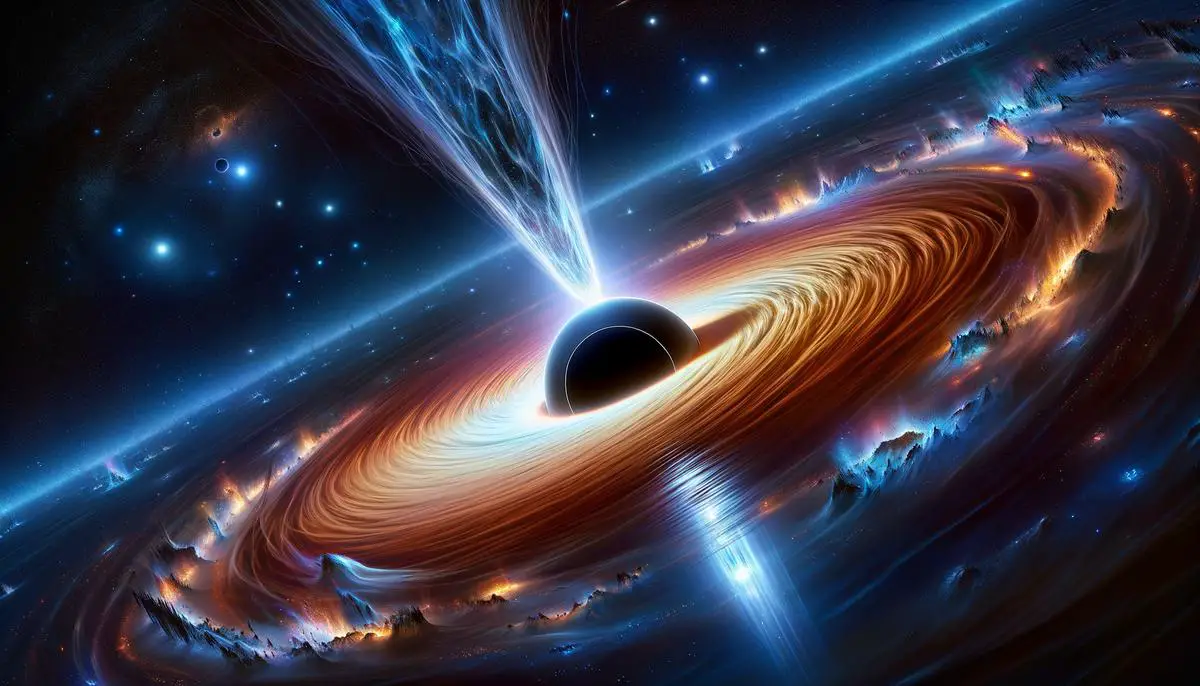
The Event Horizon
The event horizon of a black hole represents a captivating boundary in the universe. It marks the point beyond which nothing—not even light—can escape the gravitational pull of the black hole. This boundary defines the region where the escape velocity surpasses the speed of light, making anything beyond it invisible to outside observers.
The concept of the event horizon originates from Einstein's general theory of relativity, which predicts how gravity affects space-time. As a massive object like a black hole warps space-time, it creates a well so deep that beyond a certain point, the gravitational pull becomes insurmountable. This point—where the escape velocity equals the speed of light—marks the event horizon.
At the event horizon, matter and light undergo a significant transformation. As an object approaches the event horizon, it experiences intense tidal forces—the difference in gravitational pull on different parts of the object. These forces can stretch and compress the object in a process known as "spaghettification." To an external observer, the object appears to slow down and its light becomes increasingly redshifted as it nears the event horizon, eventually fading into darkness as time dilation takes effect.
From the perspective of the object falling into the black hole, however, it would continue to accelerate towards the singularity at the black hole's center without experiencing this time dilation. This dichotomy is one of the many intriguing aspects of black hole physics.
Key Characteristics of the Event Horizon:
- Not a physical surface, but a mathematical boundary
- Point of no return for anything crossing it
- Raises questions about the "black hole information paradox"
- Challenges our understanding of fundamental physics
Despite the event horizon's elusive nature, modern technology has begun to offer indirect glimpses of these regions. The Event Horizon Telescope (EHT) collaboration, which captured the first image of a black hole's shadow in the galaxy M87, provides visual evidence of the event horizon's existence1. The image shows a bright ring of light surrounding the dark shadow of the black hole's event horizon, offering a powerful confirmation of theoretical predictions.
"The event horizon stands as a crucial frontier in astrophysics. It challenges and expands our understanding of the universe, inviting deeper exploration into the laws governing space, time, and gravity."
The radiative phenomena observed near the event horizon enhance our understanding of these enigmatic boundaries. The intense gravitational field forces light to travel around the event horizon, creating intricate patterns and increasing our comprehension of how gravity warps space and time. The dynamics of matter close to this boundary, such as the energetic jets and X-ray emissions from the accretion disk, further illuminate the extreme conditions and processes that black holes generate.
Through theoretical constructs and pioneering observational techniques, we continue to uncover the mysteries beyond the event horizon, promising profound insights into the workings of our cosmos.
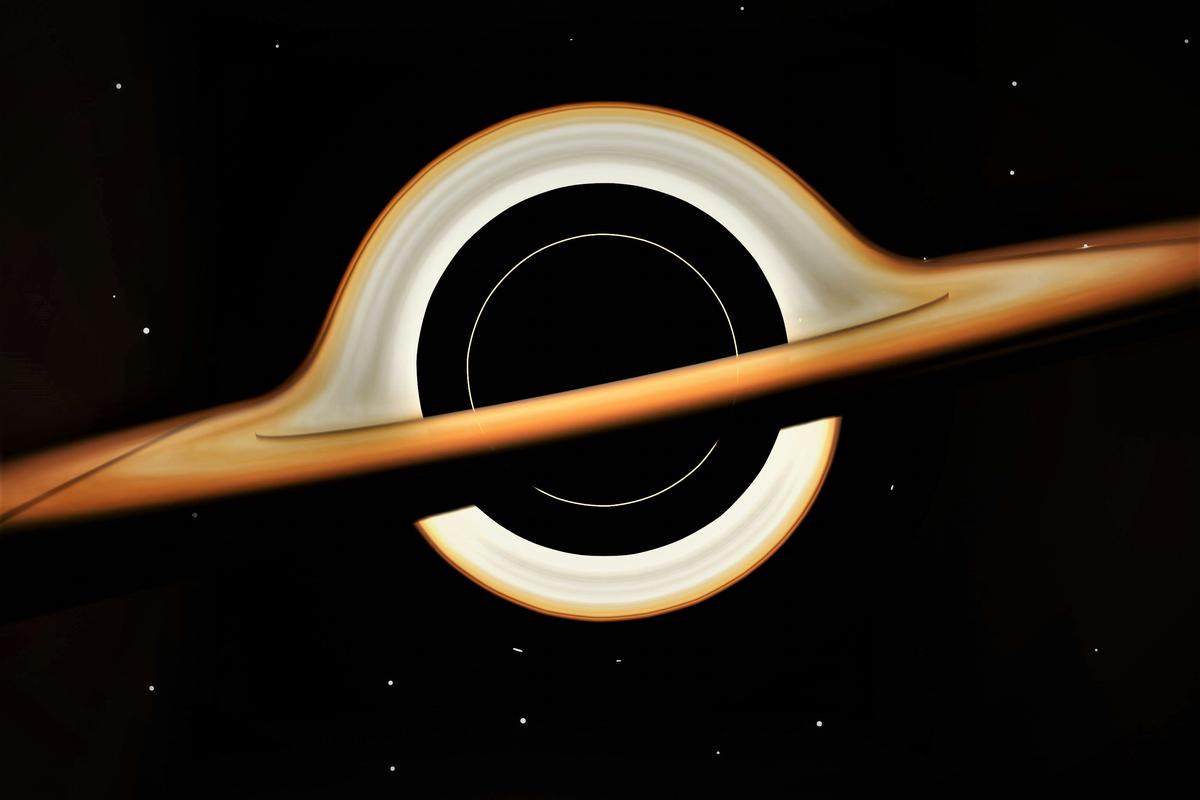
Photo by paman0744 on Unsplash
In summary, black holes are dynamic entities that significantly impact their surroundings. Their formation through stellar collapse or direct gas collapse highlights the universe's complexity. As we continue to study these enigmatic objects, our understanding of cosmic physics deepens, offering significant insights into the fabric of space-time itself.