Gravity, a force that governs the motion of planets and the fall of an apple, has intrigued scientists for centuries. From Newton's groundbreaking insights to Einstein's revolutionary theories, our understanding of gravity has continually evolved. This article explores key milestones in gravitational theory, offering a detailed look at how these concepts shape our comprehension of the universe.
Newton's Law of Universal Gravitation
Isaac Newton's Law of Universal Gravitation calculates the gravitational pull between two masses. The law states that all objects with mass attract each other with a force that depends on their masses and the distance between them. This is expressed as:
F = G * (m1 * m2) / r^2
Where:
- F is the force of gravity
- m1 and m2 are the masses of the objects
- r is the distance between their centers
- G is the gravitational constant
The force between two objects increases with mass and proximity. For example:
- If you double one object's mass, the force doubles
- Halving the distance increases the force four times
Newton's formula applies to all objects, from apples to celestial bodies.
Newton's law unified previous theories and showed that gravity is one universal force acting between all masses. Its elegance lies in its universal applicability, explaining phenomena from planetary orbits to the stability of galaxies.
On Earth, gravity keeps us grounded. The Earth's mass pulls us downward while we exert a much smaller force back on Earth. The ground pushes upward, preventing us from falling toward the center.
Newton's framework continues to assist astronomers in understanding gravitational influences. It has helped predict planetary positions, led to the discovery of new planets, and explains phenomena like gravitational lensing, where massive objects bend light from distant sources.
From the simple act of dropping an apple to the grand-scale orbits of planets, Newton's Law of Universal Gravitation profoundly impacts our comprehension of the cosmos.
Einstein's Theory of General Relativity
Einstein's Theory of General Relativity offers a deeper understanding of gravity, addressing the "how" and "why" behind gravitational forces. Unlike Newton, who described gravity as a force between two masses, Einstein proposed that gravity is a consequence of the warping of spacetime.
In Einstein's model, massive objects create dents and curves in the fabric of spacetime. Objects follow the straightest possible paths through this curved spacetime, which we perceive as the force of gravity. This idea is encapsulated in Einstein's field equations, which describe how matter and energy influence spacetime curvature, and how that curvature directs object movement.
General Relativity explained phenomena that Newton's laws couldn't, such as the slight deviation in Mercury's orbit around the Sun. It also predicted gravitational waves, ripples in spacetime caused by massive cosmic events, which were detected by instruments like LIGO in 20151.
The theory enriches our understanding of black holes, regions where spacetime is so warped that not even light can escape. It also reveals that gravity affects time, with stronger gravitational fields causing time to run more slowly—a phenomenon known as gravitational time dilation.
General Relativity's implications extend to the expansion of the universe and phenomena like dark energy. As we continue to explore the cosmos, Einstein's insights serve as a guiding principle, shaping our perception of the universe and our place within it.
Gravitational Waves
Gravitational waves, predicted by Einstein's Theory of General Relativity, are disturbances in the fabric of spacetime. These ripples are generated by colossal cosmic events such as:
- The merging of black holes
- Collisions of neutron stars
- The early moments following the Big Bang
For decades, gravitational waves remained theoretical due to their incredibly faint nature by the time they reach Earth. The Laser Interferometer Gravitational-Wave Observatory (LIGO) and its European counterpart, the Virgo interferometer, were developed to detect these minute distortions in spacetime.
LIGO's design features perpendicular arms, each several kilometers long. As a gravitational wave passes, it stretches one arm while compressing the other. This tiny fluctuation is detected by laser beams measuring the lengths of the arms with extraordinary precision.
In September 2015, LIGO detected its first gravitational wave signal, GW150914, resulting from the merger of two black holes about 1.3 billion light-years away2. This detection marked the dawn of a new era in astronomy and confirmed Einstein's century-old prediction.
Gravitational waves offer a unique way to observe the universe, complementing traditional astronomy based on electromagnetic radiation. They allow us to study phenomena invisible or obscured by interstellar dust, providing insights into:
- The formation and evolution of black holes
- Neutron star interactions
- Their role in cosmic structure
The study of gravitational waves also opens new avenues for exploring physics under extreme conditions. For instance, neutron star collisions produce elements heavier than iron, including gold and platinum. Observing these events gives insight into the processes that create such elements.
Future observatories, like the European Space Agency's Laser Interferometer Space Antenna (LISA), promise to enhance our capabilities in detecting lower-frequency gravitational waves from sources such as supermassive black hole mergers.
Gravitational waves provide a revolutionary window into the universe's most enigmatic events, transforming our understanding of gravity and spacetime dynamics. They continue to illuminate our cosmic quest, revealing the intricate dance of the universe's most extreme phenomena.
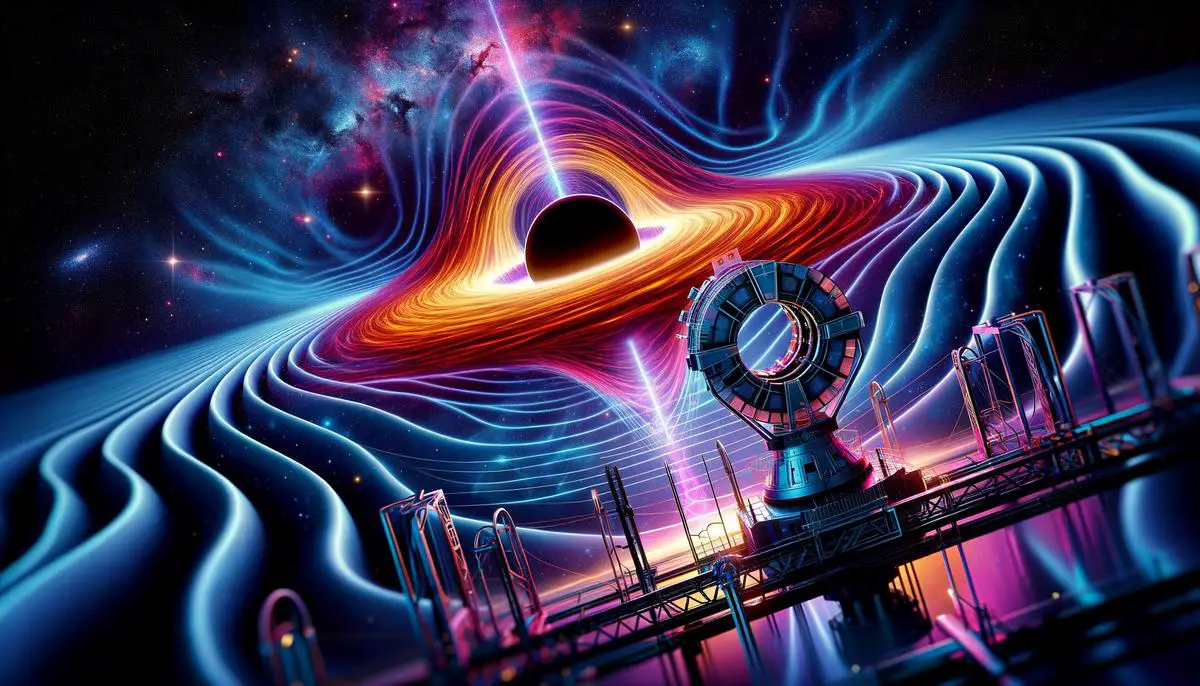
The Equivalence Principle
The Equivalence Principle, a crucial foundation in gravitational theory, emerged from Albert Einstein's work and marks a shift in our understanding of gravity. Unlike Newton's laws, which focus on gravitational force and mutual attraction between masses, the Equivalence Principle explores the nature of gravity and acceleration, leading to significant implications for General Relativity.
The principle states that gravitational and inertial forces are indistinguishable. Experiencing a force due to gravity is fundamentally the same as experiencing a force due to acceleration. For example, the force you feel in an accelerating elevator in space is identical to the gravitational pull you'd feel standing on Earth.
This equivalence led Einstein to develop the Strong Equivalence Principle: locally, the laws of physics in a freely falling reference frame are the same as in an inertial frame. This implies that it's impossible to distinguish between uniform acceleration and a gravitational field in a local environment.
Experimental Validation
The principle has been validated through numerous experiments:
- Galileo's early observations of objects falling at the same rate regardless of mass laid the groundwork.
- Ernst Mach's thought experiments and Einstein's insights set the stage for more precise empirical validations.
- The experiments of Roland von Eötvös in the early 20th century used torsion balance methods to confirm the principle to remarkable precision.
- Modern tests, such as the satellite-based MICROSCOPE mission, have validated the Equivalence Principle to unprecedented accuracy, confirming that the accelerations of different masses remain indistinguishable to within one part in 1014.
Implications for General Relativity
The Equivalence Principle is essential to General Relativity. It led Einstein to envision gravity not as a force, but as a manifestation of spacetime curvature. The presence of mass and energy warps spacetime, and objects move along the curved paths defined by this geometry.
This principle explains why objects in free fall appear weightless – they are following a natural path in curved spacetime. It also has practical implications, such as in the Global Positioning System (GPS). Satellites in orbit experience different gravitational fields than we do on the surface, and the Equivalence Principle, along with relativistic corrections, ensures accurate timekeeping for GPS functionality.
The Equivalence Principle bridges classical mechanics with modern physics and validates Einstein's revolutionary ideas. It reshaped our view of gravity from a mysterious pulling force to a fundamental aspect of spacetime geometry. Ongoing experimental efforts continue to solidify its role at the heart of gravitational theory, reminding us that the universe's workings often hinge on elegantly simple, yet profound truths.
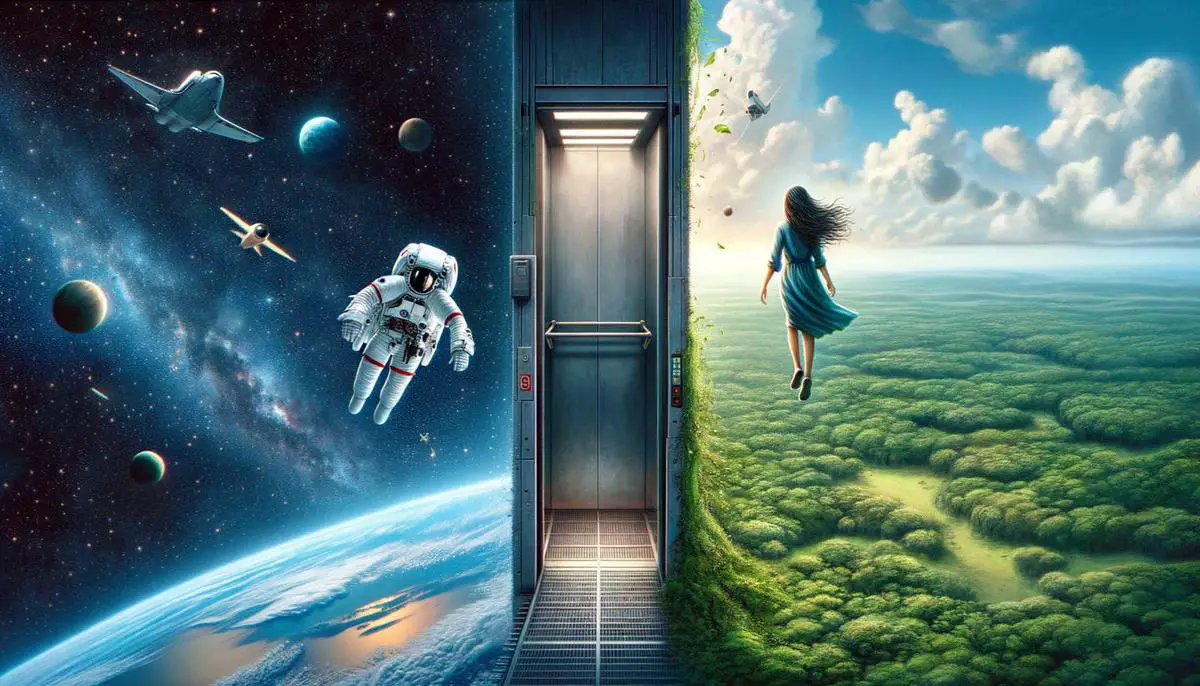
Modern Research and Discoveries
Recent years have seen significant advancements in gravitational research, thanks to technological improvements and sophisticated observational methods. These strides deepen our understanding of gravity and extend the foundational theories laid by Newton and Einstein.
Dark Matter
A key area of research is the study of dark matter—an unseen substance that exerts gravitational effects on visible matter. Its presence is inferred from its gravitational influence on galaxies and galaxy clusters. Observations reveal that galaxies spin at velocities that should tear them apart, given their visible mass, yet the gravitational pull of dark matter provides the necessary cohesion.
Modern detectors and telescopes, such as those aboard the European Space Agency's Gaia satellite, offer high-precision measurements of stellar motions and positions, accelerating our quest to map dark matter distributions. Theories on its nature range from Weakly Interacting Massive Particles (WIMPs) to axions. While direct detection remains elusive, experiments like the Large Hadron Collider and cryogenic underground detectors continue pushing the boundaries of our understanding.
Gravitational Waves
Gravitational waves represent another frontier in modern research. LIGO and Virgo's detections of these spacetime ripples not only confirmed Einstein's predictions but opened a new observational channel. These waves, generated by cataclysmic events such as black hole mergers, provide insights into previously speculative phenomena.
The detection of GW170817, a merger of neutron stars, granted insights into the formation of heavy elements, electromagnetic wave production, and the nature of extremely dense matter.1
With each detection, our understanding of the universe deepens. Future observatories like Japan's KAGRA and ESA's LISA will enhance our capabilities, broadening the spectrum of detectable gravitational waves. LISA will detect lower-frequency waves from supermassive black hole collisions, promising insights into galactic dynamics and early universe conditions.
Implications for Gravitational Theory
These advancements carry significant implications for gravitational theory. Observing matter under extreme gravitational conditions tests the limits of General Relativity. While Einstein's framework remains robust, anomalies like the accelerated expansion of the universe suggest potential modifications or new theories, such as those involving dark energy.
Dark energy, a mysterious driver behind the universe's acceleration, intertwines with gravity in ways we are only beginning to comprehend. It challenges our understanding of gravity's role on a cosmic scale, steering the universe's expansion despite gravity's tendency to pull matter together.
Modern gravitational research is a rapidly evolving field. The study of dark matter and gravitational waves extends the legacy of Newton and Einstein, propelling us into a new era of discovery. These advancements promise profound shifts in our understanding of the cosmos, challenging established theories and revealing the universe's deeper secrets. As our tools and techniques improve, so does our ability to probe gravity's enigmatic depths, uncovering new chapters in the story of the universe.
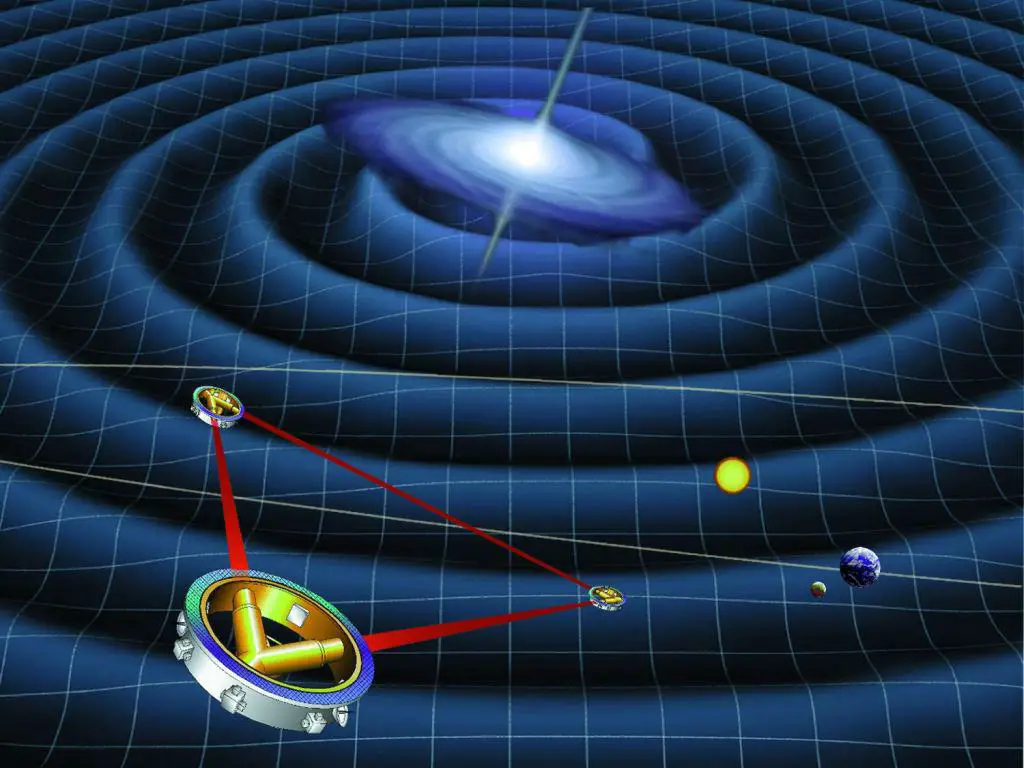