Delving into the intricacies of solar neutrinos offers a window into the sun’s core, a place where the fundamental processes that power our star unfold. This journey through the realm of subatomic particles reveals not just the mechanisms of stellar energy production but also touches upon the broader strokes of particle physics and cosmology. The narrative ahead aims to shed light on how these ghostly particles, born from nuclear reactions, travel vast distances to reach us, carrying information about the sun’s fiery heart.
Understanding Solar Neutrinos
Solar neutrinos emerge from the sun’s core, where nuclear fusion reactions transform hydrogen atoms into helium, releasing vast amounts of energy. At the heart of these processes lies the proton-proton chain, the sun’s primary energy source. This chain kicks off when two protons, or hydrogen nuclei, combine under extreme pressure and temperature, eventually forming a helium nucleus through a series of steps. Each step in this chain produces a solar neutrino, a ghostly particle that barely interacts with matter.
In addition to the proton-proton chain, the sun employs another fusion process known as the carbon-nitrogen-oxygen (CNO) cycle, albeit to a lesser extent. This cycle uses carbon, nitrogen, and oxygen as catalysts to fuse hydrogen into helium. Like its more dominant counterpart, the CNO cycle also generates solar neutrinos. Despite their different origins, both processes underscore the sun’s role as a neutrino factory.
Solar neutrinos pass through the sun and space without hardly ever touching other particles because they interact so weakly with matter. This trait makes them incredibly challenging to detect; countless neutrinos pass through the Earth and even our bodies every second without leaving a trace. To catch these elusive particles, scientists have constructed enormous underground detectors filled with substances like heavy water or gallium. A neutrino might, on very rare occasions, interact with a nucleus in these detectors, revealing its presence.
The study of solar neutrinos is not just an exercise in chasing shadows. By measuring their flux and energy, scientists can peer into the sun’s energetic core, indirectly observing processes hidden from direct view. This research tests our understanding of fundamental physics, including weak nuclear force and the intricate balance of forces that sustain stars.
While solar neutrinos were theorized in the 1960s, early experiments detected fewer neutrinos than models predicted, leading to the “solar neutrino problem.” This mystery persisted until experiments showed that neutrinos oscillate among different types, explaining the deficit. This discovery highlighted the complexities of neutrino physics and earned the Nobel Prize in Physics in 2015.
In essence, solar neutrinos serve as messengers from the sun’s interior, offering insights into stellar mechanics and fundamental particle physics. Their study bridges the gap between the atomic and cosmic scales, enhancing our grasp of the universe’s workings from the very small to the astoundingly large.
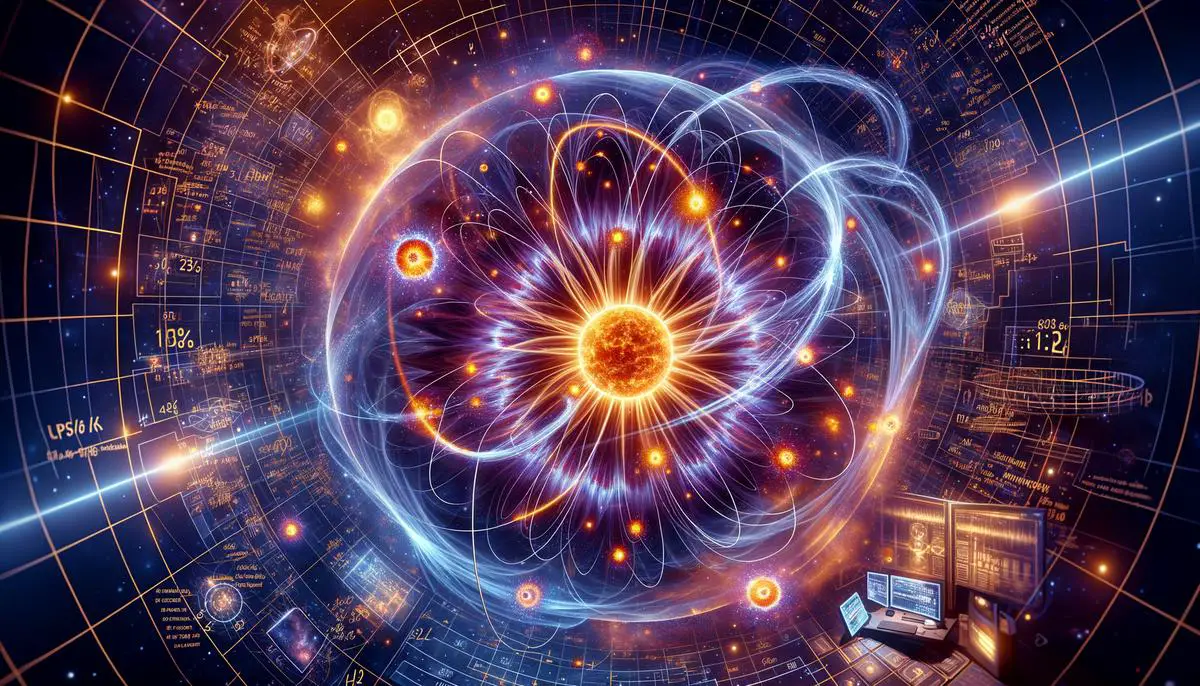
Detecting Solar Neutrinos
Diving deeper into the world of neutrino detection, the Homestake Experiment emerged as the pioneer in this realm. Spearheaded by Raymond Davis Jr., this initiative involved a large tank filled with cleaning fluid, specifically perchloroethylene, buried deep underground in a gold mine. The genius of this approach lay in its sensitivity to neutrinos, where an incoming neutrino would occasionally interact with a chlorine atom, converting it to an argon atom. This rare event could then be measured, offering direct proof of neutrino presence. Although the detection rate was lower than anticipated, it marked the first great stride in neutrino astronomy and spurred further research into this enigmatic particle.
Building on the foundation laid by the Homestake Experiment, the field of neutrino detection has since seen remarkable innovations. The Super-Kamiokande detector in Japan refined the approach by utilizing a vast tank filled with ultra-pure water, set deep beneath a mountain. This facility banks on the Cherenkov effect—where a neutrino interaction with water molecules produces a faint light, visible to the sensitive cameras lining the tank. This method’s brilliance lies not only in its ability to detect neutrinos but also in providing data on the particle’s direction, boosting our spatial understanding of where these neutrinos originate from within the sun.
Another significant development came with the Sudbury Neutrino Observatory (SNO) in Canada. SNO’s unique contribution was its use of heavy water, a rare form of water where hydrogen atoms contain an additional neutron. This setup significantly enhanced the detection capabilities, allowing the observatory to distinguish between different types of neutrinos, shedding light on the phenomenon of neutrino oscillation—where neutrinos switch between types as they travel. This groundbreaking discovery helped solve the long-standing solar neutrino problem and further unlocked the complexities of these ghostly particles.
However, capturing these elusive solar messengers is no mean feat. One of the foremost challenges is their incredibly weak interaction with matter. To shield the detectors from other subatomic particles and cosmic rays, these observatories must be located deep underground, often in former mines or beneath mountains. This location minimizes noise, enabling the faint whisper of neutrinos to be heard amid the cacophony of other particle interactions.
Yet, even within these silent chambers, the challenge persists. Neutrinos, in their billions, pass through these detectors every second, but only a handful leave a trace. Improving detection sensitivity thus becomes a paramount endeavor, requiring continual advancements in detector technology and methods to reduce background radiation further.
Despite these hurdles, the quest to detect and study solar neutrinos continues unabated. Each photon’s detection enriches our understanding of the sun’s inner workings and, by extension, the mechanisms powering our universe. Through ingenuity and perseverance, scientists unravel the mysteries of solar energy production and take steps closer to answering profound questions about the cosmos.
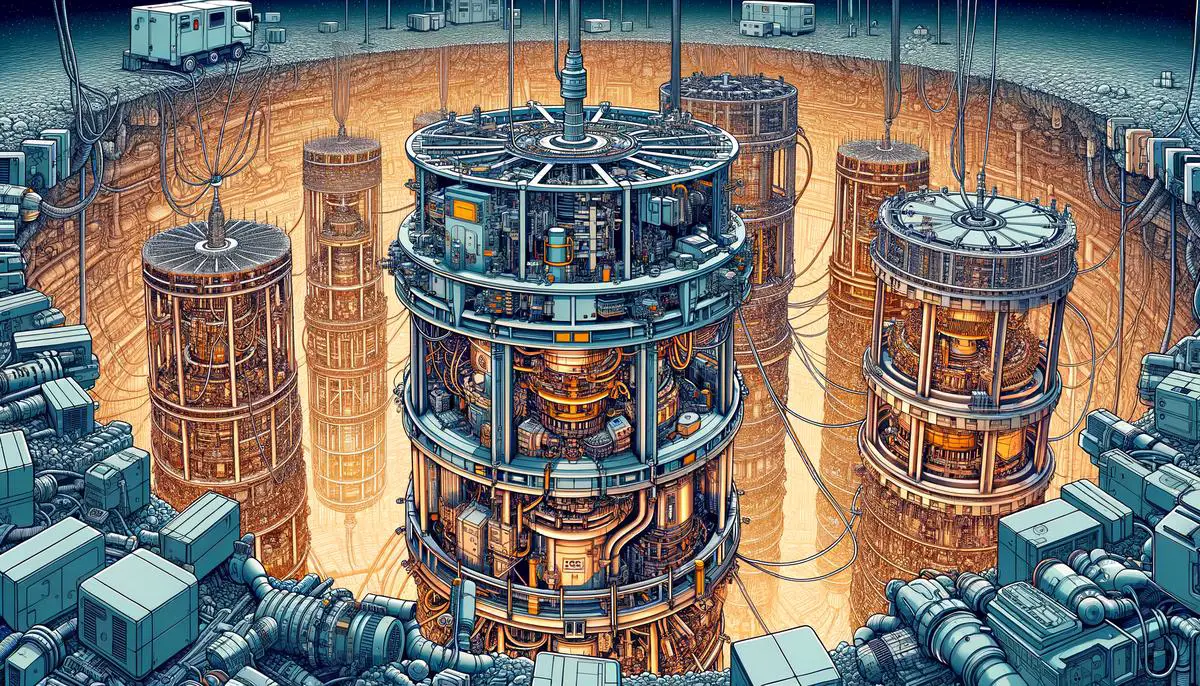
Solar Neutrinos and the Sun’s Inner Workings
Shifting the focus from the detection methods and historical context, it is crucial to explore how solar neutrinos unlock the secrets behind the sun’s powerhouse – its core. These ghostly particles serve as a gold mine of information about the nuclear reactions that fuel our closest star.
One breakthrough realization from studying solar neutrinos is the validation of the proton-proton chain reaction theory at the heart of the sun’s energy production. The exact matching of neutrino counts, as predicted by models illustrating this reaction, underscores the process taking place predominantly in solar cores. This link establishes an astounding connection between neutrinos and stellar physics, providing insights into the rate at which the sun burns its hydrogen fuel and thus, its lifespan.
Furthermore, while the proton-proton chain takes center stage, neutrino studies also hint at the presence and scale of the CNO cycle in our sun. The cycle, though secondary, is vital for massive stars and understanding its significance in our sun reveals nuances about stellar behavior across the universe.
Equally important, the solution to the solar neutrino problem introduced the world to neutrino oscillation – a phenomenon highlighting that these particles change types as they travel from the sun to Earth. This discovery not just solved a longstanding puzzle but also offered revolutionary insights into the properties of neutrinos themselves, showing these particles have mass and undergo transformations. This opened new avenues in particle physics, prompting reassessments of standard models and suggesting neutrinos may play a role in cosmic structuring beyond mere energy transfer mechanisms in stars.
Addressing these cosmic messengers, researchers witnessed first-hand the fusion processes occurring at the sun’s core. Solar neutrinos confirmed the sun operates through nuclear fusion, a concept once theoretical. Through these interactions, scientists gather details on the ratios and abundances of various elements in the sun’s core, enriching our comprehension of stellar chemistry.
Moreover, the study of solar neutrinos not only cements our understanding of how stars shine but also offers an indirect probe into probing deeper questions in cosmology and particle physics. For instance, their interactions suggest possibilities around dark matter and energy composition in the universe.
As efforts continue to refine our detection capabilities, each neutrino captured brings a wealth of data, potentially heralding new discoveries about our universe’s building blocks. The journey of studying these elusive particles underscores an enchanting narrative of intellectual curiosity leading to monumental breakthroughs in both understanding the majestic furnace powering our daily existence and the fabric of reality itself. Each neutrino detected is a storyline, narrating tales of cosmic engineering far beyond our earthly confines.
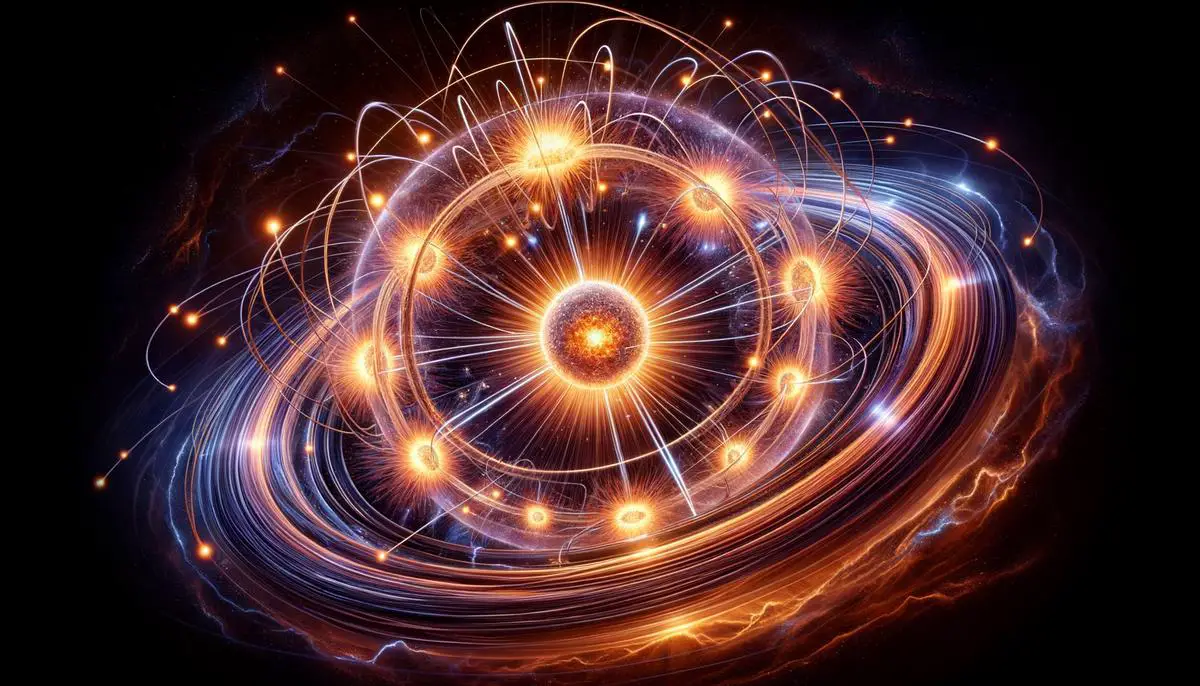
Implications of Solar Neutrino Studies
Solar neutrino research throws open the door to understanding how stars like our Sun manage their enduring glow and eventually, how they end their celestial journey. By dissecting the inner working of stars through these minuscule particles, scientists peer into the very furnaces that forge elements heavier than hydrogen, a process intriguingly mirroring alchemy at a cosmic scale. Understanding these fusion processes gives astrophysicists critical insight into the life cycle of stars, from their fiery births to their often dramatic demises as supernovae, which in turn scatters these newly formed elements across the cosmos, contributing to the cosmic cycle of birth and renewal.
Neutrinos, as part of the subatomic particle family, play a significant role in the Standard Model of particle physics, yet their mass remains an anomaly not accounted for by this model. The discovery of neutrino oscillations challenged long-standing theories, suggesting that neutrinos have mass, however slight. This revelation prompted a broader inquiry into the physics beyond the Standard Model, potentially including unverified interactions or undiscovered particles. The quest to reconcile these observations with current theories pushes the boundaries of particle physics and stokes the fires of inquiry into phenomena like dark matter and energy, which dominate the universe’s structure but remain enigmatic.
The peculiar behavior of solar neutrinos also enhances our understanding of the interplay between forces at a quantum level, particularly the weak nuclear force—one of the four fundamental forces in the universe. The weak force, responsible for radioactive decay and nuclear fusion, thus becomes more tangible through the lens of neutrino studies, allowing physicists a clearer understanding of these processes that sculpt the observable universe.
Amid these breakthroughs lies the promise of neutrino astronomy, forging a new avenue for observing astronomical phenomena beyond electromagnetic radiation. By tracing neutrinos that are birthed in supernovae or the nuclear reactions in other stars, astronomers anticipate uncovering stellar secrets that light cannot unfurl. This nascent field might one day provide a window into the workings of neutron stars and black holes, objects whose densities and gravitational pull warp the space around them so intensely that traditional astronomy’s reach is limited.
Moreover, solar neutrino studies offer an invaluable toolkit for probing into the early universe’s conditions shortly after the Big Bang. Neutrinos, barely interacting with matter and thus streaming through space unimpeded, carry pristine information from the universe’s infancy. By studying these elusive particles, cosmologists hope to unravel the conditions and processes that led to the formation of cosmic structures, from galaxies to clusters and superclusters, providing insights into the universe’s formation and evolution.
As solar neutrino research progresses, it intertwines astrophysics and particle physics more deeply, promising answers to some of the most profound questions about our universe’s building blocks and the forces that weave them into the vast cosmic tapestry. Each discovery propels our quest further, turning once speculative theories into tangible realms of investigation and offering glimpses into the universe’s future through the vantage of its most elusive particles.
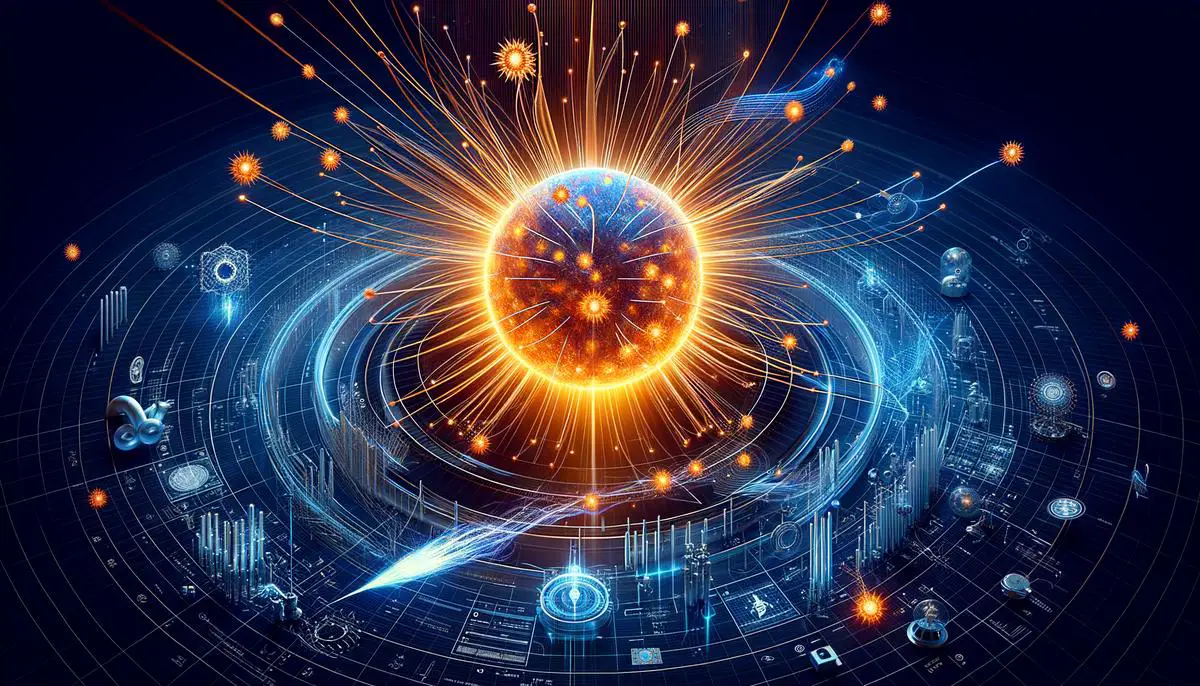
In conclusion, solar neutrinos stand as silent witnesses to the sun’s internal machinations, offering us a glimpse into the nuclear reactions that fuel our closest star. Through their study, we not only refine our understanding of stellar processes but also push the boundaries of particle physics and cosmology. Each neutrino detected is a testament to the sun’s relentless energy production, serving as a beacon that guides our quest for knowledge about the universe’s most fundamental aspects.