Gravity, a fundamental element of our universe, asserts its pull on every speck of matter, shaping cosmic structures and defining the very fabric of existence. As a fundamental natural force, gravity helps the Earth maintain its orbit around the Sun, compels the ocean tides, and allows life to exist and evolve. Grasping the mechanics of gravitational pull demands an understanding of brilliant theories posited by minds like Sir Isaac Newton and Albert Einstein. This comprehension starts with the principles of gravitation and progresses towards complex concepts interacting with the curvature of space-time, and finally touching upon the effects and applications of gravity in our everyday life and beyond.
Basic Principles of Gravitation
Understanding the Indomitable: Foundational Laws of Gravitational Force
Gravity, an inescapable concept threaded into our daily lives through the irresistible pull of the Earth beneath our feet, has intrigued astronomers, physicists, and philosophers alike for centuries. From Sir Isaac Newton’s deductions in the 17th century to the groundbreaking revelations by Albert Einstein in the 20th, the foundational laws governing gravitational force have evolved, branched, and fused our comprehension of this cryptic force of nature.
Sir Isaac Newton, through his keen observational acumen and meticulous empiricism, propounded the Universal Law of Gravitation. The law postulates that any two objects in the universe, irrespective of the distance, attract each other with a force that is directly proportional to the product of their masses and inversely proportional to the square of the distance between them. It is a law so fundamental and deeply ingrained in our understanding of physics that it permits us to predict the behavior of heavenly bodies and plays a pivotal role in technological developments such as satellite launches and GPS functionality.
However, the turn of the 20th century marked a paradigm shift. The towering intellect of Albert Einstein challenged and ultimately refined our understanding of gravity with his theory of General Relativity. This theory, laudably daring and stunningly profound, analyzes gravity not as a force in the customary sense, but rather as a distortion in the fabric of space-time caused by massive objects. In essence, matter tells space-time how to curve, and curved space-time directs how matter moves.
Einstein’s illustration of gravity encapsulates the concept of objects in motion. He indicated that an object in constant linear motion will continue to follow a straight path in space-time unless acted upon by external forces. However, in the vicinity of a massive body (e.g., a planet), space-time stretches or warps, causing the object to deviate from its straight-line trajectory and begin following a different path – often an elliptical or hyperbolic path that we perceive as gravitational attraction.
It is important to mention that Newton’s law isn’t erased by Einstein’s theory; instead, General Relativity encompasses it. For near-Earth calculations and many practical applications, Newton’s law suffices and is more convenient. Yet, General Relativity accurately accounts for the gravitational effects not only where Newton’s law still works but also where it starts to falter, like the precise tracking of planet Mercury’s orbit and the perception of light bending around massive cosmic bodies.
Hence, in the cryptic wonderland of gravity, we observe two laws harmoniously coexisting. Newton’s Universal Law of Gravitation, a pure and elementary interpretation of gravitational interaction, coalesces with Einstein’s Theory of General Relativity, a profoundly intricate view of gravity as distortions in the fabric of the cosmos, to deliver a comprehensive understanding of gravitational force. Both laws, fundamentally integral in their unique paradigms, continue to ignite a beacon of comprehension in the mysterious domain of gravity, underpinning groundbreaking discoveries and innovations in contemporary science.
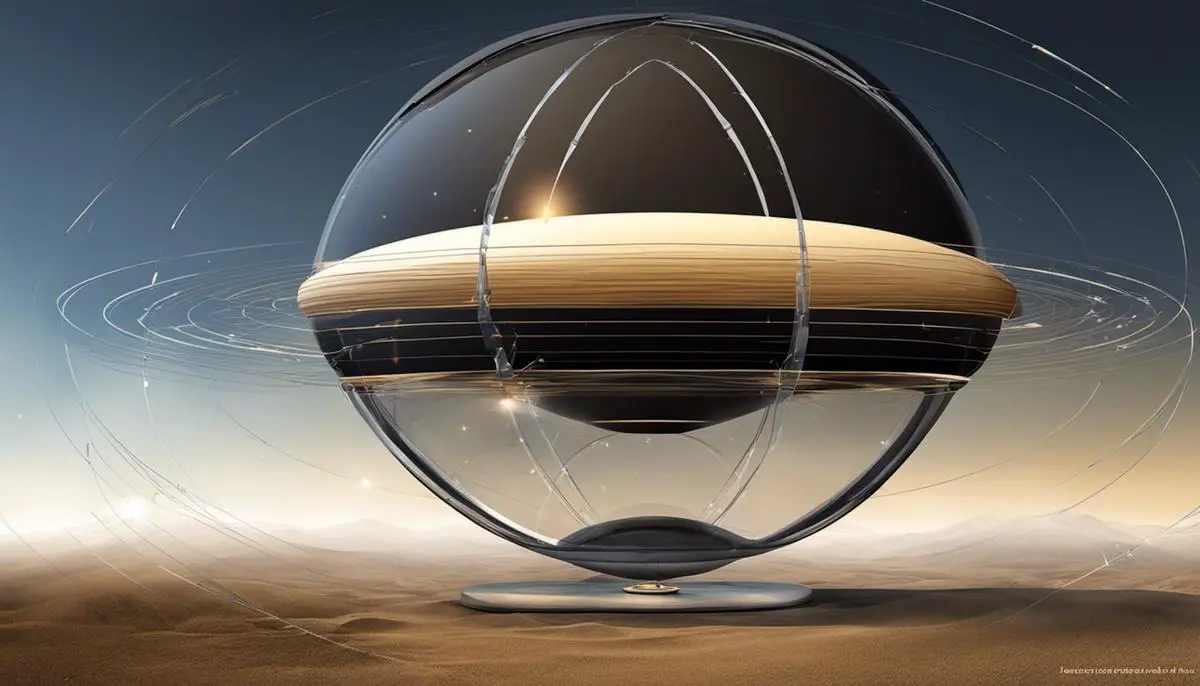
Earth’s Gravitational Field
The complexity and intrigue of Earth’s gravitational field remains as a captivating focus in the realm of physics. It exercises an omnipresent and profound influence, from governing celestial alignments to affecting technology embedded intricacies. Embracing the theoretical foundations postulated by Newton and Einstein, this article flourishes into the more tangible implications of Earth’s gravitational pull.
Undeniably, it is the gravitational force that secures the Earth in its orbital procession around the Sun, an association dictated by the mass of the celestial bodies and the distance separating them. In turn, it commands the lunar orbit around Earth. This celestial choreography accounts for a multitude of earthly phenomena, inclusive of tides.
Tides are the measurement of the constant interaction that takes place between Earth and its neighboring celestial bodies. They result from the gravitational pull the Moon exerts on Earth’s surface, a pull stronger on the side nearest the Moon, leading to a bulge of water or “high tide”. However, as Earth rotates, areas move into and out of this tidal bulge, which manifests as the cyclic pattern of rising and falling sea-levels.
Expanding this understanding to a larger scale, Earth’s gravitational sphere of influence extends into space, creating a protective barrier against solar radiation and preserving our atmosphere. This realm, known as the magnetosphere, demonstrates the implication of Earth’s gravitational field on the planet’s habitability and space weather patterns, which subsequently affect satellite functionality and telecommunications.
Furthermore, Earth’s gravitational field is not uniform; it subtly varies across the planet’s surface due to the irregular distribution of mass. It is dually influenced by the size and density of physical features, including mountains, deep sea trenches, and interior geological structures. Collectively, these anomalies provide critical insights into geophysical and climatological processes.
For instance, by measuring minor fluctuations in Earth’s gravity, scientists can monitor water storage fluctuations in areas susceptible to drought— a pioneering advancement in the struggle against climate change. Concurrently, a comprehensive understanding of the gravitational field aids in refining GPS technology, improving navigation accuracy for myriad applications, ranging from aviation to satellite imagery.
Finally, in the enthralling field of astrophysics, Earth’s gravity plays an indispensable role. Understanding the fine balance of speeds and gravitational pull is critical for space missions and satellite launches. This intricate dance between kinetic and potential energy is the core concept behind escape velocities, or the speed at which an object must travel to break free of a planet’s gravitational force.
In sum, the essence of Earth’s gravitational field transcends the foundational laws postulated by Newton and Einstein, burgeoning into direct implications affecting a diversity of phenomena. This unceasing exploration of our countless interactions with Earth’s gravitational force serves as a testament to our desire to understand the complex world we inhabit. Our continual pursuit to unravel the intricacies of this potent force are of paramount importance in addressing present and future challenges, within our world and beyond.
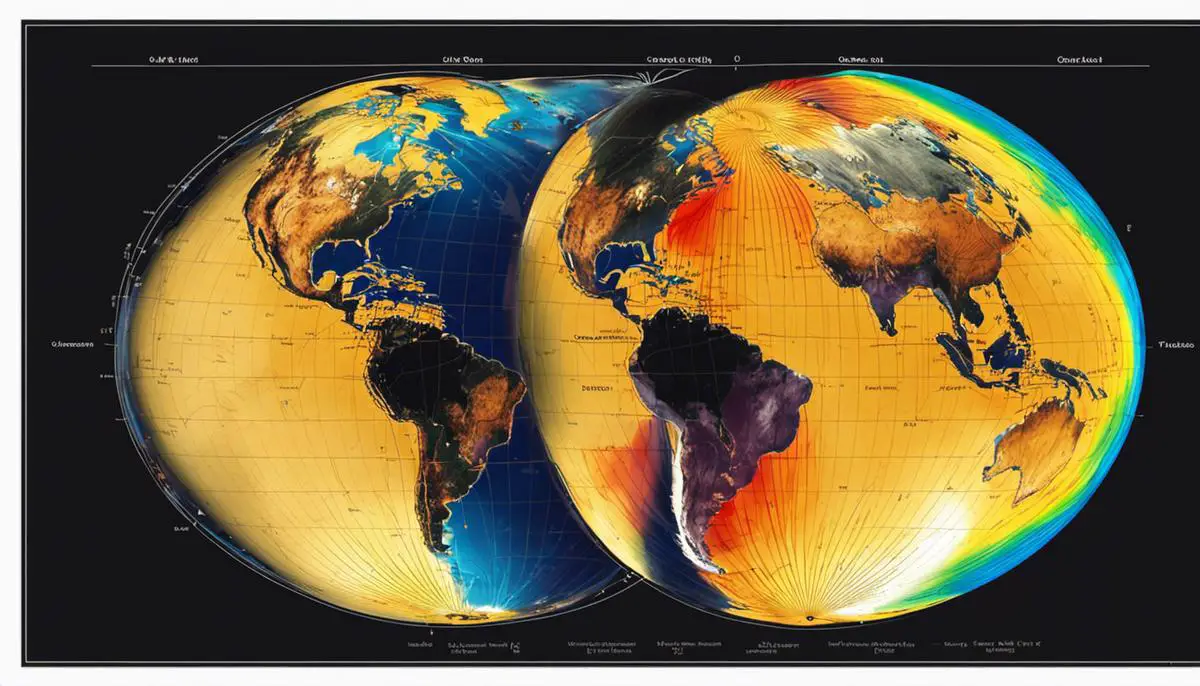
Gravity and Space-Time
Pivoting forward, contemporary physicists are extending the profound implications of gravity in our world that go beyond Einstein and Newton’s foundational theories, ascending into groundbreaking arenas such as gravitational waves and black holes.
Allegedly envisioned by Albert Einstein, gravitational waves, or ripples in the fabric of space-time generated by accelerating massive objects, remained hypothetical until the Laser Interferometer Gravitational-Wave Observatory (LIGO) confirmed their existence in 2016. The detectability of gravitational waves has delivered an innovative instrument for astronomers, facilitating a new method of observing cosmic phenomena, like colliding black holes or neutron stars, that were previously oblivious, thereby signifying gravity’s role in shaping and bending space-time.
The investigation into the nature of black holes, those enigmatic celestial bodies with gravitational forces so strong that not even light can escape, has grown into a captivating subdomain of astrophysics. The gravitational pull at the chilling event horizon is extreme to such an extent that it distorts space-time into peculiar configurations, where the fabric of the universe stretches to infinity, and time essentially halts, creating a veritable abyss in the framework of reality.
While these groundbreaking revelations are entrancing, they are also instrumental in challenging our understanding and provoking further research into gravity’s role in the distortion of space-time. For example, black holes have become subjects of interest for physicists testing the limits of general relativity, where it seems to break down and a more comprehensive theory, such as quantum gravity, may be required.
Similarly, in the enigmatic field of quantum mechanics, gravity has yet to find its place. Despite general relativity’s unimagined success in describing the macroscopic world, the quantum domain remains elusive. The thriving search for a unified theory, such as String Theory or Loop Quantum Gravity, underscores the determination to reconcile both regimes and comprehend gravity in the quantum arena, where space-time is no longer smooth but perhaps quantized at incredibly minute scales.
Gradually, the intrinsic connection between gravity and space-time distortion is prompting physicists toward a more sophisticated comprehension of the universe. Physicists are tirelessly working on unifying the realms of large (general relativity) and small (quantum mechanics), thereby inching closer to uncover the true nature of gravity and its critical role in warping the reality of space-time.
The ongoing unscrambling of these hidden implications of gravity harbors the potential of transformative breakthroughs in our grip on the fabric of the cosmos and the fundamental laws that govern it. Acknowledging gravity’s role in the distortion of space-time, one can only marvel at the gravity of the subject at hand. This fascination, propelled by the profound curiosity and unremitting dedication of researchers, will undeniably continue to glean novel insights on the saga of space-time and beyond. The pursuit to comprehend gravity’s enigma epitomizes the ceaseless quest for knowledge that propels modern physics, underscoring its pivotal role in our ceaseless probe into the boundless vertex of the cosmos.
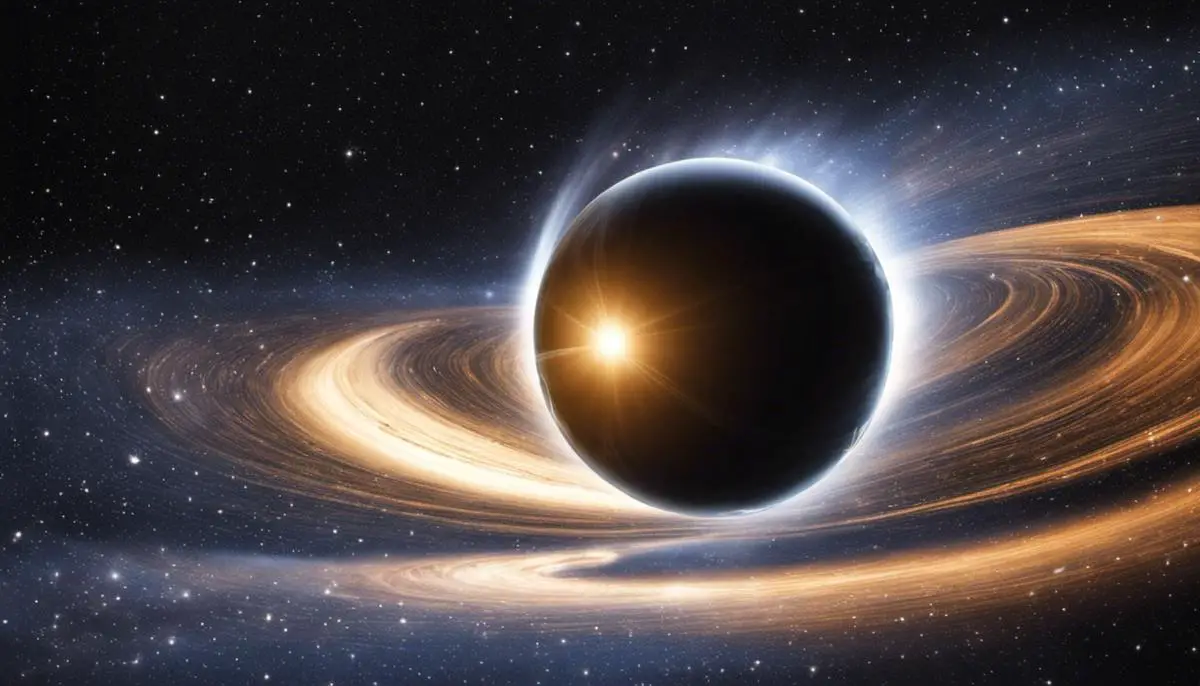
Effects and Applications of Gravitation
The dynamic interplay of life and technology under Earth’s gravitational influence is a topic of entirely engrossing depth. Having understood its fundamentals and implications per Newton’s and Einstein’s revolutionary insight, the focus now turns toward understanding the myriad ways in which gravitational force impacts daily existence for Earth’s inhabitants, as well as for our advancements in technology.
Let us first examine the effects of Earth’s gravitational pull on life forms. The evolution of life on Earth has been, in essence, a grand experiment under relatively uniform conditions of gravity. All terrestrial organisms, from plants standing tall to humans walking upright, have adapted inherent structural strengths to resist gravity. Bones, for instance, adapt to the demands of gravity by growing stronger and denser where the strongest pressures are felt. Even the very rhythm of life is affected; the circadian rhythms synchronizing biological and behavioral processes to a roughly 24-hour cycle owe their existence to Earth’s gravitationally-controlled rotation.
Sundry physiological systems in organisms display adaptive strategies to gravitational force. Heart rate and blood pressure in humans, for instance, and the transport of water and solutes in plants are modulated with implicit acuity to the pull of gravity. Research conducted on astronauts has shed light on some striking effects caused by human exposure to reduced gravity. These range from muscular atrophy and bone density loss to changes in spatial perception and cognitive function.
In the realm of technology, it is hard to overstate gravity’s role. Aviation and the launch of satellites into orbit are perhaps the most direct examples where the gravitational pull of Earth must be surmounted. However, grasp the impact of gravity’s influence extends across a swath of technological developments. From influencing the pressure differences that drive our weather systems, and hence climate modeling, to factoring into designs of large-scale structures such as skyscrapers and bridges — gravitational force remains a constant consideration.
Consider GPS technology, a system that relies on precise time measurement. The clocks on GPS satellites experience slightly less gravitational pull than clocks on Earth. As per Einstein’s theory of relativity, time moves slower where gravity is strong. Therefore, the satellite clocks are faster by about 38 microseconds per day. This minuscule discrepancy, if uncorrected, would result in significant navigational errors.
Progresses in the realm of gravity research have also brought fascinating discoveries like gravitational waves. Detected by the Laser Interferometer Gravitational-Wave Observatory (LIGO), these ‘ripples’ in space-time provide a novel way to study celestial phenomena such as merging black holes and neutron stars.
Black holes, objects with such strong gravity that nothing, not even light, can escape, further deepen the enigma of gravitational forces. The space-time distortion near a black hole’s event horizon is a testament to the power of gravitational pull, pushing our understanding of physics to its limit.
In the pursuit to integrate gravity into quantum mechanics, a singular challenge persists. A lack of experimental evidence for quantum effects of gravity hampers any definitive progress. Undeterred, researchers continue in the quest for a unified theory that could reconcile the seemingly irreconcilable – gravity and quantum physics.
As such, the influence of Earth’s gravitational pull is immanent and far-reaching, anchoring life and driving technology in ways more profound than we often appreciate. The quest for understanding gravity, in its full complexity, remains one of the noblest pursuits in the scientific world — for with comprehensive knowledge of gravity, one grasps the very sinews that hold the cosmos together.
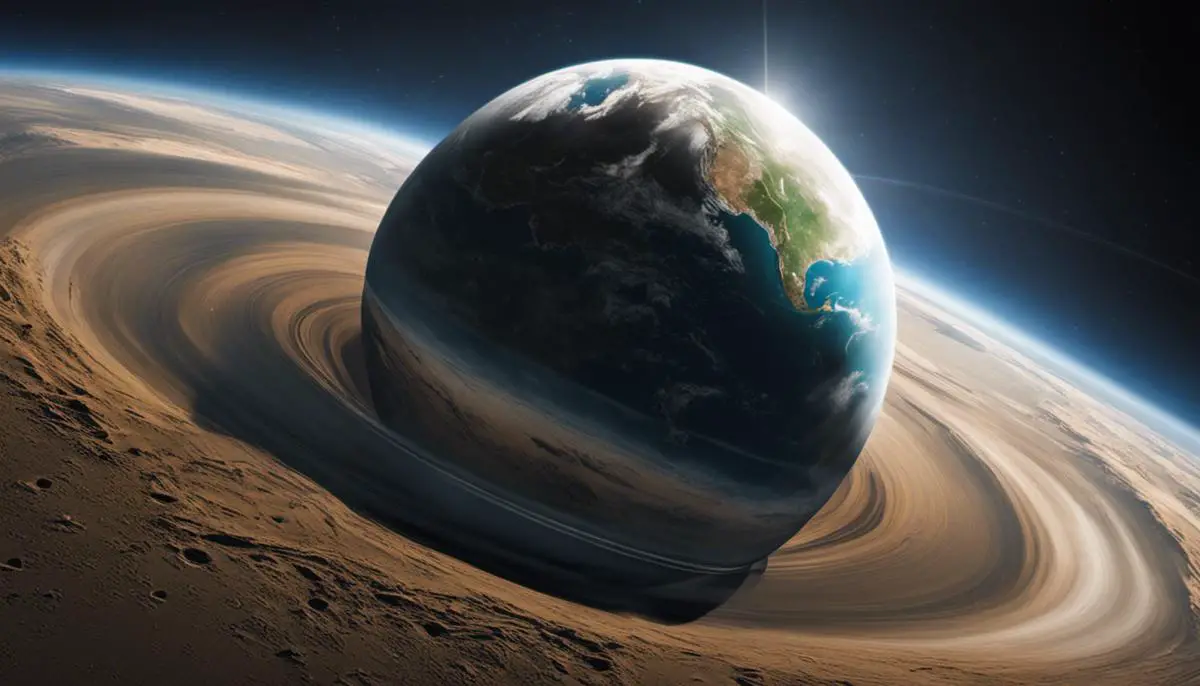
Understanding gravity unravels not only the mystery of the falling apple but also inspects its influence on our complex world. From determining the spherical shape of the Earth and influencing climate patterns, to enabling satellite orbits and fostering advances in technology and research – physics’ silent workhorse never ceases to shape reality. Mastery of this knowledge has space-time proportions as we ground ourselves on Earth, while eyeing the infinite cosmos. As we orbit in the grasp of this unseen force, it serves as a reminder of our humble existence in the face of the cosmic order dictated by gravity.